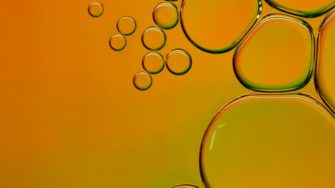
Chemical innovations changing our world
Theoretical chemists’ daily work influences our understanding of the way the world works. From improving efficiencies in manufacturing processes to categorising new compounds and materials and helping other research areas extract useful knowledge from data, practical applications of theoretical chemistry will help us solve many future problems facing our society.
Playing a key role in physical chemistry, theoretical chemistry seeks to develop theories and explanations to understand the structure, movement and reactions of molecular systems in the absence of an experiment. By developing and applying novel computational and analytical techniques, theoretical chemists can predict molecular structure, dynamics, bonding, chemical reactivity and physical properties to provide ongoing experiments with new insights.
Associated schools, institutes & centres
Impact
Our research covers a broad spectrum of theoretical and computational chemistry, principally within the biological and physical chemistry domains. Much of our work is predicated on the idea that if you cannot simulate a chemical process in detail, then you cannot claim to understand that process in detail. We work on both the development of novel theoretical approaches as well as the application of a range of techniques to “interesting” problems in modern chemistry. Irrespective of the level of theoretical novelty, our work requires large-scale computational investigation—either as an end unto itself for more applied work or to allow validation of new and modified theory.
Our research covers:
- accurate and scalable chemical quantum dynamics methodology
- simulations of gas-surface chemistry and heterogeneous catalysis
- materials defect engineering for new colossal permittivity dielectric materials
- energetic materials and propellants
- understanding the fundamental nature of quantum mechanical reality
- complex behaviours in soft condensed matter.
Competitive advantage
Our work is primarily computational and is performed at a variety of scales including:
- a few atoms treated with full quantum mechanical detail (including all quantum mechanical effects at the Schrödinger equation level for nuclei as well as electrons).
- DFT modelling of condensed phase systems and catalytic surfaces.
- statistical mechanical and continuum treatments of soft matter, ionic liquids, and biological membrane features.
Some of our recent work on interpretations of quantum mechanical electronic structure represents the first serious progress with truly novel fundamental insight since the early days of quantum mechanics.
Successful applications
- New quantum dynamics methodology using Gaussian basis functions for the nuclear wave function.
- Fundamental insights into the electronic structure associated with the bonding in molecules, including extracting banana bonds, lone pairs and spin unpairing from high-level ab initio quantum chemistry calculations.
- Breakdown of the “constant surface normal velocity” approximation in combustion.
- Engineered defects for dielectric materials used in manufactured electronic components.
-
We have long-established and close research links to complementary groups within:
-
Y. Liu, P. Kilby, T.J. Frankcombe and T.W. Schmidt, “The electronic structure of benzene from a tiling of the correlated 126-dimensional wavefunction,” Nature Commun., 11 (2020) 1210.
H. Mai, T. Lu, Q. Sun, R. G. Elliman, F. Kremer, T. Duong, K. Catchpole, Q. Li, Z. Yi, T. J. Frankcombe and Y. Liu, “High performance bulk photovoltaics in narrow-bandgap centrosymmetric ultrathin films,” Mater. Horizons, 7 (2020) 898.
T. Murakami and T.J. Frankcombe, “Non-adiabatic quantum molecular dynamics by the basis expansion leaping multi-configuration Gaussian (BEL MCG) method: multi-set and single-set formalisms,” J. Chem. Phys., 150 (2019) 144112.
Y. Liu, P. Kilby, T. J. Frankcombe and T. W. Schmidt, “Calculating curly-arrows from ab initio wavefunctions,” Nature Commun., 9 (2018) 1436.
Q. Sun, D. Cortie, S. Zhang, T.J. Frankcombe, G. She, et al., “The formation of defect-pairs for highly efficient visible light catalysts,” Adv. Mater., 2017 (2017) 1605123.
T.J. Frankcombe, “Explicit calculation of the excited electronic states of the photosystem II reaction centre,” Phys. Chem. Chem. Phys., 17 (2015) 3295.
W. Hu, Y. Liu, R.L. Withers, T.J. Frankcombe, L. Norén, A. Snashall, M. Kitchin, P. Smith, B. Gong, H. Chen, J. Schiemer, F. Brink and J. Wong-Leung, “Electron-pinned defect-dipoles for high-performance colossal permittivity materials,” Nature Mater., 12 (2013) 821.
Stenberg S; Stenqvist B; Woodward C; Forsman J, 2020, 'Grand canonical simulations of ions between charged conducting surfaces using exact 3D Ewald summations', Physical chemistry chemical physics: PCCP, vol. 22, pp. 13659 – 13665
Vo P; Lu H; Ma K; Forsman J; Woodward CE, 2019, 'Local Grand Canonical Monte Carlo Simulation Method for Confined Fluids', Journal of Chemical Theory and Computation, vol. 15, pp. 6944 – 6957
Sun D; Forsman J; Woodward CE, 2017, 'Molecular Simulations of Melittin-Induced Membrane Pores', The Journal of Physical Chemistry B, vol. 121, pp. 10209 - 10214