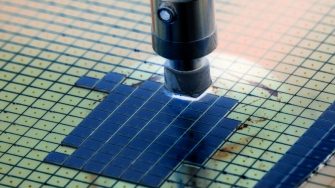
In the Semiconductors for Energy Applications Group, we use a combination of computational and experimental methods to develop new materials, heterostructures and nanostructures for renewable energy applications, particularly photoelectrochemical and photocatalytic hydrogen generation, solar cells and batteries.
Research program
Solar hydrogen generation
One of the critical barriers to increased use of renewable energy sources is their intermittency. To overcome this barrier, an efficient means of storing the energy must be found.
One approach is to store the energy in the form of chemical energy in clean fuels. This can be done with photoelectrochemical (PEC) or photocatalytic water splitting - a practical approach to generating hydrogen using energy from sunlight. This process requires a photoactive semiconducting material, that can absorb sunlight to produce electron-hole pairs, which are used to drive the water splitting redox reaction.
While there are known semiconductors that can be used for water splitting (e.g. TiO2), they all have deficiencies that limit their solar-to-hydrogen conversion efficiency. These include wide band gaps, that limit how much of the visible part of the solar spectrum can be absorbed, and poor surface catalytic properties.
In our group, we are focusing on materials of complex compositions - e.g. ternary and quarternary compounds, or binary compounds combined together in the form of solid solutions or heterostructures. These complex compositions provide greater capacity to tune the material properties and hence performance.
We use computational methods (e.g. DFT) to understand the structure-property relationships of such complex compositions and structures and hence design optimised materials, in combination with experimental work to synthesise, characterise and test the performance of materials.
We focus on optimising band gaps and understanding surface catalytic processes in order to tune the surface properties to minimise the reaction activation barriers.
Catalysis
We also collaborate with researchers in a range of fields, providing computational analysis to aid interpretation of experimental results.
In particular, we work with researchers from the UNSW Particles and Catalysis Research Laboratory to understand the surface chemistry and reactivity of catalysts.
Batteries and photovoltaics
Our group also uses computational methods to study semiconductors for solar cells and materials for Li- and Na-ion batteries, particularly ion transport and electrode-electrolyte interactions.
Group news
- Congratulations to Hongyang (Jason) Ma and Xinxin Lu, who have recently been awarded their PhDs. Hongyang is now a post-doctoral researcher in the Quantum Materials & Device Research Group, UNSW School of Physics.
- Welcome to Owen Bowdler, Jason Wooi and Thibault Cosseron De Villenoisy who have recently joined the group as PhD students.
Recent publications
- Uncovering Atomic-Scale Stability and Reactivity in Engineered Zinc Oxide Electrocatalysts for Controllable Syngas Production (Advanced Energy Materials, 10(28), p.2001381)
- Enhancement of CeO2 Silanization by Spontaneous Breakage of Si–O Bonds through Facet Engineering (J. Phys. Chem. C 2020, 124, 4, 2644–2655)
- DFT Study of Methanol Adsorption on Defect-Free CeO2 Low-Index Surfaces (ChemPhysChem, 20(16), pp.2074-2081)
- ZnS-GaP Solid Solution Thin Films with Enhanced Visible-Light Photocurrent
- Light-Induced Formation of MoOxSy Clusters on CdS Nanorods as Cocatalyst for Enhanced Hydrogen Evolution
- GaP–ZnS Multilayer Films: Visible-Light Photoelectrodes by Interface Engineering
- DFT study of various tungstates for photocatalytic water splitting
- Light-Induced Synergistic Multidefect Sites on TiO2/SiO2 Composites for Catalytic Dehydrogenation
ZnS-GaP Solid Solution Thin Films with Enhanced Visible-Light Photocurrent
Reprinted with permission from ACS Appl. Energy Mater. 2021 (10.1021/acsaem.1c01733). Copyright 2021 American Chemical Society.
Light-Induced Formation of MoOxSy Clusters on CdS Nanorods as Cocatalyst for Enhanced Hydrogen Evolution
Reprinted with permission from ACS Appl. Mater. Interfaces 2020, 12, 7, 8324–8332. Copyright 2020 American Chemical Society.
GaP–ZnS Multilayer Films: Visible-Light Photoelectrodes by Interface Engineering
Reprinted with permission from J. Phys. Chem. C 2019, 123, 6, 3336–3342. Copyright 2019 American Chemical Society.
DFT study of various tungstates for photocatalytic water splitting
Reprinted with permission from Phys. Chem. Chem. Phys., 2020,22, 1727-1737. Copyright 2020 Royal Society of Chemistry.
Light-Induced Synergistic Multidefect Sites on TiO2/SiO2 Composites for Catalytic Dehydrogenation
Reprinted with permission from ACS Catal. 2019, 9, 3, 2674–2684. Copyright 2019 American Chemical Society.
Our team
Bosi Huang
PhD Student - Ternary tungstate photoelectrodes for solar water splitting
Louis Oppong-Antwi
PhD Student - CuS/ZnS heterostructures for solar water splitting
Owen Bowdler
PhD Student - Exploiting dynamic stimuli to promote photoelectrochemical organic oxidation using bismuth ferrite
Rebecca Dawkins
PhD Student - Screen-printed reference electrodes
rebecca.dawkins@student.unsw.edu.au
Thibault Cosseron De Villenoisy
PhD Student - Stabilising the monoclinic phase of ZrO2 through doping
Jason Wooi
PhD Student - Understanding and optimising electrode/electrolyte interfaces in Na-ion batteries