- UNSW
- ...
- Our schools
- Chemical Engineering
- Our research
- Potential PhD projects
- Home
- About us
- Study areas
-
Our research
- Research areas
-
Research centres
- Australian Carbon materials centre
- ARC Training Centre for Advanced Technologies in Food Manfacturing
- ARC Training Centre for Global Hydrogen Economy (GlobH2E)
- Algae & Organic Matter Laboratory
- Australian Centre for Nanomedicine
- Centre for Advanced Macromolecular Design
- Particles & Catalysis Research Group
- Process Control Group
- UNESCO Centre for Membrane Science & Technology
- ProMO Group
- Cordelia's Research Lab
- Postgraduate Research – PhD and MPhil
- Potential PhD projects
- Open Lab Week
- Student life
- Alumni & industry
- News & events
- Home
- About us
- Study areas
-
Our research
Research centres
- Australian Carbon materials centre
- ARC Training Centre for Advanced Technologies in Food Manfacturing
- ARC Training Centre for Global Hydrogen Economy (GlobH2E)
- Algae & Organic Matter Laboratory
- Australian Centre for Nanomedicine
- Centre for Advanced Macromolecular Design
- Particles & Catalysis Research Group
- Process Control Group
- UNESCO Centre for Membrane Science & Technology
- ProMO Group
- Cordelia's Research Lab
- Student life
- Alumni & industry
- News & events
Potential PhD projects
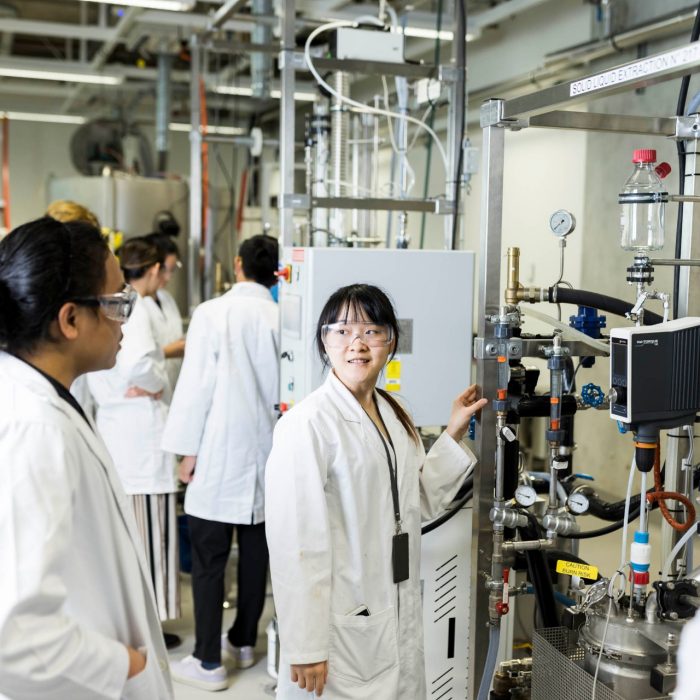
Bioengineering and health
-
Supervisor:
Dr Peter R. Wich, Email, opens in a new window | School of Chemical Engineering, UNSW
www.wichlab.com, opens in a new window
Project summary
Nature’s polymers, such as polysaccharides and proteins show a remarkable versatility as multifunctional materials. They can be easily modified with the toolkit of bioorganic chemistry and are particularly attractive because of their degradability and biocompatibility. Since they are structurally well-defined biopolymers they can be used for the formation of multifunctional nanomaterials, for example for the delivery of therapeutic drugs or being part of advanced molecular machines. The open PhD project will focus on the preparation of biopolymer-based nanomaterials using various chemistry and nano/biotechnological approaches.
Key techniques (projects can be adjusted based on interest and experience): organic chemistry, biochemistry, bioconjugation methods, nanoparticle preparation, bio assays, cell culture
Environment: The project will be undertaken the Wichlab in the School of Chemical Engineering which is part of the Centre for Advanced Macromolecular Design (CAMD, www.camd.unsw.edu.au, opens in a new window) and the Australian Center for NanoMedicine (ACN, www.acn.unsw.edu.au, opens in a new window).
For further information about the Wichlab, please visit:
-
Supervisor:
Dr Peter R. Wich, Email, opens in a new window | School of Chemical Engineering, UNSW
www.wichlab.com, opens in a new window
Project summary
Nature’s polymers, such as polysaccharides and proteins show a remarkable versatility as multifunctional materials. They can be easily modified with the toolkit of bioorganic chemistry and are particularly attractive because of their degradability and biocompatibility. Since they are structurally well-defined biopolymers they can be used for the formation of multifunctional nanomaterials, for example for the delivery of therapeutic drugs or being part of advanced molecular machines. The open PhD project will focus on the preparation of biopolymer-based nanomaterials using various chemistry and nano/biotechnological approaches.
Key techniques (projects can be adjusted based on interest and experience): organic chemistry, biochemistry, bioconjugation methods, nanoparticle preparation, bio assays, cell culture
Environment: The project will be undertaken the Wichlab in the School of Chemical Engineering which is part of the Centre for Advanced Macromolecular Design (CAMD, www.camd.unsw.edu.au, opens in a new window) and the Australian Center for NanoMedicine (ACN, www.acn.unsw.edu.au, opens in a new window).
For further information about the Wichlab, please visit:
-
Supervisor:
Professor Guangzhao Mao, Email | UNSW School of Chemical Engineering
Project summary
Nanowire sensors promise to be the next generation building block for ultra-sensitive and ultra-selective detection of chemical species. However, transitioning nanowire sensors from the laboratory into economical devices has proven problematic. Current manufacturing processes do not scale well in terms of precision, consistency, and yield, and are too expensive to justify commercial development. There is a critical unmet need for enabling technologies for precision manufacturing of nanosensor devices. The overall goal of the project is to synthesize nanowire crystals of charge-transfer salts (CTS-NWs) directly on electronic substrates by seed-mediated electrocrystallization for precision manufacturing of nanosensor devices. We hypothesize that CTS-NWs will nucleate and grow on the nanoelectrodes and their size and morphology will be controlled by those of the electrodes. The specific objectives are: (1) to evaluate the effect of electrode size on the electrocrystallization of CTS-NWs; (2) to study the electrocrystallization of CTS-NWs using faceted metal nanoparticles as nucleation seeds; and (3) to demonstrate precision manufacturing of CTS-NW sensors using seed-mediated electrocrystallization on patterned nanoelectrodes.
Key techniques: seed-mediated nucleation, nano-electrochemistry, microelectrodes, charge-transfer salt crystallization, nanomaterials characterization, chemical sensors.
Environment: you will work with Professor Mao and her experienced team of researchers in a highly collaborative and interdisciplinary environment.
For further information please contact Professor Mao., opens in a new window
Clean energy technologies
-
Supervisor
Dr. Dipan Kundu, Email, opens in a new window
Project summary
It would not be an overstatement to say that lithium-ion batteries have changed our world dramatically. After revolutionizing the portable electronics sector, their rapid penetration into electric vehicles (EV) and renewable storage market is projected with much optimism. However, the state-of-the-art batteries, developed for electronics application, falls short both in terms of performance and safety features required for EV and stationary storage applications. Among several alternatives, solid-state batteries and aqueous rechargeable batteries have exciting prospects. Yet, there are many challenges – from materials, chemistry, and mechanism related shortcomings to stability, scalability, and processability related issues. These issues aggravate further when parameters beyond the lab scale are considered. The available research projects in our team seek to overcome those challenges through evaluation of science underpinning the devices, discovery of new materials, in-depth assessment of their electrochemistry and a better understanding of structure-performance relationships, under practical operational conditions. Key areas of focus are (i) understanding, development, and optimization of solid-state alkali metal (Li & Na) batteries and battery components using solid electrolytes, (ii) development of novel inorganic and polymeric solid electrolytes with superior Li+/Na+ conductivity, (iii) aqueous rechargeable Zn (anode) batteries for large-scale (grid-storage, renewable, home) stationary storage applications, and (iv) operando electrochemical analyses of battery materials and novel operando cell development.
Key techniques: Solid-state and materials chemistry – advanced synthesis and characterizations, diverse physicochemical analysis, electrochemistry, operando electrochemical studies, battery testing, cell development, and scale-up of lab-scale electrochemical performance.
Environment: The project will be undertaken in the Electrochemical Storage Engineering Laboratory ( ESEL) in the School of Chemical Engineering at UNSW.
For further information, please visit our team page.
-
Supervisor
Dr Nicholas Bedford, Email, opens in a new window | School of Chemical Engineering, UNSW
Project summary
The properties and behaviors of every single material is directly dictated by the chemistry of its atoms and the arrangement of these atoms in 3D space. The importance of structure and how it relates to properties is universally acknowledged in the field of catalysis. Yet despite the recognized importance of the so-called “structure-function” relationship, methods for systematically understanding the nature of these reactive structural motifs are often ignored. As a result, much of the new developments in catalysts are usually serendipitous, impeding technological progress due to a lack of rational design rules for making new materials. Our research group aims to course-correct for status-quo materials development by combining creative synthetic techniques with new methodologies for identifying and subsequently engineering atomic-scale catalytic structural motifs in nanoscale catalyst using advanced characterization techniques. Synchrotron radiation characterization is central to the efforts in this project, wherein a student is expected to participate in experiments at synchrotron facilities worldwide.
Key techniques: Materials synthesis techniques are flexible to the student’s skillsets and interests. Our group specialized in bio-inspired techniques, multi-metallic nanomaterials and 2D layered materials, but are eager to expand into other areas. Catalytic applications are also flexible, although the group is currently focused on electrocatalytic reactions geared toward alternative energy constructs. Although previous synchrotron expertise is not required, a desire to participate in experiments, process data, and build nanoparticle structure models is a must.
Environment: The project will be undertaken with the Bedford Research Group, opens in a new window in the School of Chemical Engineering which is part of the Particle and Catalysis Research Group., opens in a new window
For further information, please visit:
-
Supervisor
Prof. Liming Dai, Email, opens in a new window | UNSW School of Chemical Engineering
Project summary
Owing to the high earth-abundance, eco-friendliness, high electrical conductivity, structural tunability at the atomic/morphological levels, good stability under harsh conditions, and free from metal dissolution and poisoning, carbon nanomaterials have become promising advanced metal-free catalysts for high-performance renewable energy technologies.
The recent research and development of carbon-based metal-free electrocatalysts (C-MFECs) have opened up new research areas for multifunctional electrocatalysis of oxygen reduction reaction (ORR) in fuel cells, ORR and oxygen evolution reaction (OER) in metal-air batteries, and OER and hydrogen evolution reaction (HER) for overall water-splitting. This open PhD project will focus on the new fundamentals governing the multifunctional metal-free carbon electrocatalysis, and the breakthroughs that enable C-MFECs as metal-free multifunctional electroctrocatalysts for various energy-related reactions even beyond the ORR, OER, and HER. This project covers the structure design and synthesis, multifunctional electrocatalysis mechanism, and potential applications of the C-MFECs in clean energy conversion and storage, chemical conversion, and environmental protection.
Key techniques and contribution: (projects can be adjusted based on interest and experi-ence) Carbon nanomaterial synthesis, chemical vapor deposition growth of carbon nanotubes/graphene, and device assembling for energy conversion and storage. This project is designed to gain a deep understanding of metal-free carbon-based electrocatalysis and to develop original synthetic methods for the development of metal-free advanced carbon-based electrocatalysts of practical significance.
Environment: All facilities necessary for the research are available in my group and at the School of Chemical Engineering, which will support the smooth progress of the project. The fabrication and characterization tools, such as chemical vapor deposition, sputter coater, plasma reactor, electrochemical testing unit, scanning electron microscope (SEM), thermogravimetric/differential analysis (TG/DTA), Ultraviolet/Visible spectroscopy (UV/vis), Photoluminescence spectroscopy (PL), Fourier transform infrared spectroscopy (FTIR), gas chromatography (GC) are available in my lab. Transmission electron microscope (TEM), X-ray photoelectron spectroscopic spectrometer (XPS), X-ray diffraction (XRD), Atomic force microscopy (AFM), Attenuated Total Reflectance (ATR), Raman spectroscopy, Nuclear Magnetic Resonance (NMR) Spectroscopy, Inductively coupled plasma (ICP), X-ray fluorescence spectrometry (XRF), scanning probe microscope (SPM), and high-performance liquid chromatography (HLC) are readily available at UNSW.
-
Supervisor
Dr Liming Dai, Email | UNSW School of Chemical Engineering
Project summary
Carbon-based metal-free catalysts are extensively exploited as promising heterogeneous electrocatalysts for a variety of reactions related to renewable energy technologies. Through proper chemical doping, structural engineering and morphological tuning to tailor the properties of carbon nanomaterials, remarkable electrocatalytic activities have been achieved for oxygen reduction reaction, overall water splitting, and carbon dioxide reduction. However, it is still a big challenge to understand the active site structure and detailed catalytic mechanism. Therefore, identifying the origin of electrochemical activity on these carbon-based catalysts will be critical to designing superior catalytic materials and further improving their performance. The open PhD project will focus on the preparation of structure well-defined carbon materials, as well as the use of in-situ and ex-situ experimental techniques to determine the active site structures of carbon-based metal-free catalysts.
Key techniques: (projects can be adjusted based on interest and experi¬ence) Chemical vapor deposition, micro-device fabrication, electrochemical measurements, electron or scanning probe microscopy, spectroscopic tools for in-situ characterization.
Environment: The fabrication and characterization tools, such as chemical vapor deposition, sputter coater, plasma reactor, electrochemical testing unit, scanning electron microscope (SEM), thermogravimetric/differential analysis (TG/DTA), Ultraviolet/Visible spectroscopy (UV/vis), Photoluminescence spectroscopy (PL), Fourier transform infrared spectroscopy (FTIR), gas chromatography (GC) are available in my lab. Transmission electron microscope (TEM), X-ray photoelectron spectroscopic spectrometer (XPS), X-ray diffraction (XRD), Atomic force microscopy (AFM), Attenuated Total Reflectance (ATR), Raman spectroscopy, Nuclear Magnetic Resonance (NMR) Spectroscopy, Inductively coupled plasma (ICP), X-ray fluorescence spectrometry (XRF), scanning probe microscope (SPM), and high-performance liquid chromatography (HLC) are readily available at UNSW.
-
Supervisor
Scientia Prof Rose Amal, Email, opens in a new window | Dr Ali Jalili, Email,, opens in a new window UNSW School of Chemical Engineering
UNSW Particle and Catalysis Research Group website, opens in a new window
Project summary
Ammonia (NH3) production via the Haber–Bosch process was one of the key achievements of the 20th century, enabling quadrupling the production of agricultural products and acting as a preventive measure to stop world hunger. It is estimated that between a third to a half of the world population would suffer starvation if ammonia-based fertilisers were not available. Ammonia is also an excellent indirect hydrogen-storage material as it is more energy efficient to produce, store, and deliver hydrogen as ammonia than as compressed and/or cryogenic hydrogen. Ammonia is also seen as an eco-friendly energy source for a sustainable energy future as it can be synthesised directly from atmospheric nitrogen and does not produce CO2 during burning. However, the industrial production of ammonia is very energy intensive (2% of global energy consumption), requiring high temperature (500◦C) and pressure (in excess of 500 atmospheres), and very pure raw materials; it is also eco-destructive (creating more than 900 million tonnes of CO2 by-product per year), costly and requires considerable plant infrastructure. As such, there is an immediate need to develop alternative green and cost-effective processes for ammonia production. Ammonia can be produced at room temperature by electrochemical synthesis. However, all of the state-of-art systems have common limitations such as slow kinetics of the transformation, expensive electrolytes, high pressure & temperature (to increase the kinetics), poor selectivity, low conversion rate and low Faradaic efficiency. Building on this foundation, atomically thin two-dimensional (2D) materials such as graphene will be studied over this PhD project for an efficient electrochemical reduction of nitrogen to ammonia.
Research environment
The student working on this project will be working in the Particles and Catalysis Research Laboratory (PARTCAT), a leading catalysis research group in Australia lead by Scientia Professor Rose Amal and jointly supervised by Dr Ali Jalili. To achieve this goal, the group also actively collaborates with different groups such as Prof Doug Macfarlane group in Monash University. The students will have access to state-of-the-art experimentation facilities, mentoring opportunities and fun and nurturing working environment to gain necessary expertise facilitating their career in industry or academic research.
Top up scholarship is available. Please inquire within.
-
Supervisor
Dr Priyank Kumar, Email, opens in a new window | Scientia Prof Rose Amal, Email,, opens in a new window UNSW School of Chemical Engineering
Particle and Catalysis Research Group website, opens in a new window
Project summary
Plasmonic nanostructures offer a platform to utilize sunlight to carry out chemical reactions. However, the efficiency and selectivity of such catalysts remain poor. The group uses density functional theory (DFT) and time-dependent DFT (TDDFT) methods to understand these issues and design efficient plasmonic catalysts. Calculations are performed in experimental collaboration with the PARTCAT group at UNSW, led by Prof. Rose Amal.
Research environment
The student working on this project will be working in the Particles and Catalysis Research Laboratory (PARTCAT), a leading catalysis research group in Australia lead by Scientia Professor Rose Amal and also the computational materials group in the school of chemical engineering at UNSW Sydney, led by Dr. Priyank V. Kumar , aims to develop material solutions in the areas of renewable energy, water and health-care through the application of atomistic computational techniques. To achieve this goal, the group actively collaborates with different experimental groups across UNSW and around the globe. The students will have access to state-of-the-art experimentation facilities, mentoring opportunities and fun and nurturing working environment to gain necessary expertise facilitating their career in industry or academic research.
Top up scholarship is available. Please inquire within.
Food science and technology
-
Supervisors
Associate Professor Alice Lee, alice.lee@unsw.edu.au, opens in a new window, Dr Rona Chandrawati, rona.chandrawati@unsw.edu.au, opens in a new window and Professor Guangzhao Mao, guangzhao.mao@unsw.edu.au, opens in a new window | UNSW School of Chemical Engineering
Project summary
Oral immunotherapy (OIT) has been shown to be a promising approach to tackle established peanut allergy. However, studies to date using standard allergen extracts or naïve foods have failed to generate high rates of long-term tolerance and have had high and unpredictable side effects, including anaphylaxis. These issues have impacted on food allergy-OIT translations into clinical practice. A safer option for allergic children and patients with high sensitivity would be epicutaneous immunotherapy. Epicutaneous immunotherapy involves rapid uptake of allergenic molecules by skin antigen-presenting cells and transports them to regional lymph nodes.
This PhD project aims to develop a new allergen delivery system with a controlled release for non-invasive, safer and more effective delivery of food allergens to the skin immune system via epicutaneous immunotherapy.
Key techniques
Organic chemistry, biochemistry, biotechnology, immunology, immunochemistry, cell culture
Environment
The project will be undertaken the School of Chemical Engineering within Food and Health Cluster.
-
Supervisors
Associate Professor Alice Lee, alice.lee@unsw.edu.au, opens in a new window | UNSW School of Chemical Engineering, Associate Professor Debbie Palmer, opens in a new window (Telethon Kids Institute, University of Western Australia)
Project summary
Breastfeeding is one of the factors that could influence food allergy development. These include potentially anti-allergic immune properties in the milk, the possibility that prolonged breastfeeding may delay allergen introduction, but also the presence of antibodies within breast milk that may combine with food antigens to induce tolerance. Yet the role of maternal immune responses in desensitisation and tolerance induction is still poorly understood.
As part of the BENEFIT and PrEggNut projects, this Ph.D. project aims to investigate the role of maternal immune responses in peanut and egg allergy desensitisation (or peanut and egg allergy development), including developing an analytical method to detect and quantify peanut and egg peptides in breastmilk at trace levels.
Key techniques
Protein chemistry/ biochemistry, biotechnology, mass spectrometry, bioinformatics, immunology, immunochemistry, cell culture
Research environment
The Trials will be conducted in Perth at the Joondalup Health Campus and Telethon Kids Institute at the Perth Children’s Hospital and analysis of samples will be conducted the Food and Allergy Research Group in the School of Chemical Engineering.
-
Supervisors
Associate Professor Alice Lee, alice.lee@unsw.edu.au | UNSW School of Chemical Engineering; Professor Dianne Campbell, Children’s Hospital at Westhead
Project summary
Oral immunotherapy (OIT) is a promising approach to tackle established peanut allergy. However, studies to date using standard allergen extracts or naïve foods have failed to generate high rates of long-term tolerance following cessation of therapy and have had high and unpredictable side effects, some of which include anaphylaxis. These issues have impacted on food allergy-OIT translations into clinical practice.
Using adjuvants to enhance regulatory effects of OIT and to drive transient desensitisation to more sustained tolerant responses has shown promising results. We currently have a three-arm, placebo-controlled RCT underway at the Children’s Hospital at Westmead, which examines the potential of short-chain fatty acids (SFCA) as an adjuvant in peanut OIT; the OPIA study.
The Ph.D. project aims to investigate the specific mechanisms of action of the therapy and the ability to predict responses to therapy. It will focus on developing an understanding of pivotal allergen-specific antibody responses across the course of the IT, and identify factors that can predict response to therapy.
Key techniques
Protein chemistry/ biochemistry, molecular allergology, biotechnology, bioinformatics, immunological techniques, cell culture
Environment
OPIA trial is being conducted in the Children’s Hospital at Westmead and analysis will be conducted in the Food and Allergy Research Group in the School of Chemical Engineering. This project provides an opportunity for a student to interact and work with a diverse group of project investigators.
-
1. Project title: Replacing Traditional Cell Culture Supplements with Plant-Based Alternatives for Cellular Agriculture.
Supervisor
Prof Johannes le Coutre | School of Chemical Engineering | UNSW
Project summary
Traditional biological supplements used in mammalian cell culture include fetal calf serum, opens in a new window and expensive, high-carbon footprint, recombinant proteins, opens in a new window that are used to promote the growth rates of cultured cells, opens in a new window. One of the goals of the global cellular agriculture, opens in a new window field is to identify alternative compounds with attractive carbon footprints to these traditional additives. This project will focus on using plant-based materials as the source of these compounds. The PhD project will aim to combine the chemical engineering processes of plant material extraction, with the biochemical methods of primary mammalian cell culture to achieve this goal.
2. Project title: Identifying Alternative Biomaterials to be used as Scaffolds for In Vitro 3D Muscle Cell Culture.
Supervisor
Prof Johannes le Coutre | School of Chemical Engineering | UNSW
Project summary
The field of cellular agriculture, opens in a new window and in vitro meat production is an emerging solution to growing global concerns regarding resource use, opens in a new window by traditional agriculture practices. A major obstacle for this research is the identification of low carbon biomaterials that can provide a suitable support network for cultured muscle cells, opens in a new window to form a 3D structure. This project will test the potential of various plant-based materials to provide attachment sites and mechanical support to muscle cells in culture.
3. Project title: Controlling Growth and Differentiation of Animal Muscle Cells without Genetic Modification.
Supervisor
Prof Johannes le Coutre | School of Chemical Engineering | UNSW
Project summary
The field of cellular agriculture, opens in a new window and in vitro meat production is an emerging solution to growing global concerns regarding resource use, opens in a new window by traditional agriculture practices. A major obstacle for this technology is the control of growth and differentiation rates of meat forming muscle cells, opens in a new window in culture, opens in a new window. Traditional biological supplements used in cell culture include expensive, high-carbon footprint fetal calf serum, opens in a new window and recombinant proteins, opens in a new window. One of the goals of the global cellular agriculture field is to identify alternatives to these additives. It is of critical importance that these additives do not rely on genetic modification, opens in a new window of the muscle cell itself as we aim to use cultured meat for human consumption. This project will identify non-traditional compounds to control muscle cell growth and differentiation in culture.
Key techniques of the projects
- Mammalian cell culture.
- Live-cell imaging using the Incucyte S3 system
- Recombinant protein production.
- Bioartificial muscle production.
- Novel mechanical and chemical-based extraction of plant materials.
- Flow cytometry., opens in a new window
- Confocal microscopy., opens in a new window
- Investigation of intracellular signalling pathways.
For further information please contact Prof Johannes le Coutre.
- Mammalian cell culture.
-
Supervisor
Dr Robert Driscoll, Email, opens in a new window | UNSW School of Chemical Engineering
Project summary
Extrusion is a food processing technology which can do a wide range of conventional processes all within one piece of equipment. An extruder can shape, expand and texture a product. It can also mix, cook and create pillow structures, very popular with consumers. One thing it can’t do is adequately dry, and so many extruders are combined with some form of dryer equipment, such as fluidisation. Little research has been done into optimal combinations of extrusion / drying. The main variables are the initial product composition (especially moisture), the type of product being made (formed, textured or expanded), energy parameters such as power required per unit mass produced (also called specific mechanical energy), speed of rotation, degree of heating, torque and others. We would like to develop a better understanding of the interaction between the two processes so that we can control them better in commercial practise.
Research environment
The PhD student will work in the Food Drying Group, using a Brabender KETSE 20/40 laboratory extruder recently purchased for the ARC Industry Hub.
Novelty and contribution
Twin screw extruders have a complex geometry which is difficult to effectively model, even using high-end techniques such as CFD, simply because of the wide changes in product properties as it changes from a powder to a dough. As a result, little has been done in this area, but its importance to the food industry is very high. Instead of a trial and error approach, we are looking for an intermediate approach, involving practical experimentation plus semi-empirical modelling such as a TOEM approach to improve our understanding in this area.
Expected outcome
This work will allow industry projects to be developed involving extrusion, so is of great practical importance. In addition development of a new approach to extruder modelling has the prospect of allowing publication.
-
Supervisory team
Dr Francisco Trujillo, Email | UNSW School of Chemical Engineering
Dr PJ Cullen, Email | UNSW School of Chemical Engineering
Project summary
Radio frequency electric fields (RFEF) processing is a new emerging food processing technology that can inactivate photogenic microorganisms in liquid foods such as apple juice, orange juice and apple cider at moderate sub-pasteurization temperatures. Unlike pasteurisation, where inactivation is achieved thermally, the lethal effect of RFEF is mainly due to high intensity electric fields. Microbial inactivation under RFEF is very fast compared to conventional thermal processing based on conduction and convection. This allows designing a very efficient process operated at lower temperatures than traditional heat processing. The problem of heat processing is that high temperature destroys heat sensible compounds. RFEF is able to obtain microbiologically safe foods retaining higher nutritional value and exhibiting better organoleptic properties due to lower processing temperature and time.
The RFEF mechanism of inactivation is electroporation, which is the formation of pores in the cell membrane causing the release of intracellular liquid. However, the mechanism is not completely elucidated yet. Besides, scaling up RFEF process is challenging due the complex interaction between the electric field, food material, fluid flow and the heat generated due to ohmic and dielectric heating. Hence, the aim of this research project is to better understand the complex physical and microbiological phenomena governing RFEF processing of liquid foods.
Research environment
The PhD student will have the opportunity to work within the food processing group and be part of the ARC training centre.
Novelty and contribution
This is a promising new research area at the frontier of novel food processing technologies. It is part of the search of alternatives to traditional heat processing technologies to achieve food microbiological safety at lower processing temperatures therefore enhancing nutritional value while preserving its organoleptic properties.
Expected outcome
It is expected that the successful candidate will participate in international conferences and will publish his/her work in high impact factor journals. This work will expand the capabilities of the student to work in the industry of to continue a research or academic career.
-
Supervisor
Dr Francisco Trujillo, Email, opens in a new window | UNSW School of Chemical Engineering
Project summary
CSIRO Agriculture and Food now welcomes applications for a PhD top-up scholarship to develop ultrasound based technologies to promote oil recovery in vegetable oil separation process. CSIRO Breakthrough Processing Group now welcomes Expressions of Interest for Top-up Scholarships to PhD students with chemical or food engineering, chemistry or physics background wanting to join an exciting, interdisciplinary team in the priority area of Megasonics.
-
Supervisor
Prof Cordelia Selomulya | UNSW School of Chemical Engineering
Dr Yong Wang | UNSW School of Chemical Engineering
Sian Henson | Jacobs Douwe Egbert R&D
Dr. Borja Corrochano | Jacobs Douwe Egbert R&D
Project summary
Powdered products, often produced via spray drying, extend shelf life and ease of transport, and are convenient for consumers. When fat is present in the feed emulsions to spray drying, surface fat tends to be over-represented on the final powders with fat migration taking place during the droplet atomisation process. The presence of surface fat could induce off-flavour due to oxidation, poor wettability, and reduced shelf life of the powders.
Scholarship
JACOBS DOUWE EGBERTS (JDE) Postgraduate Scholarship is available for an outstanding full-time postgraduate candidate to undertake an industry-focused research project. The PhD project aims to understand the relationship between powder properties in relation to those of the feed emulsions and other process conditions, including pre-treatment options. The functions and interactions between proteins, fats, polysaccharides, and other small molecules, affecting colloidal stability of the emulsions and under different conditions, will be identified to improve fat encapsulation of the final product.
The project provides an excellent opportunity for an outstanding full-time postgraduate candidate to solve a real-world challenge in food powder processing. The project will develop new value-added products and innovate current processing methods, in a close partnership with one of the leading beverage companies in the world, Jacobs Douwe Egberts (JDE),, opens in a new window the maker of Moccona and many other famous tea and coffee brands.
In addition to research work conducted at the School of Chemical Engineering, the PhD candidate will have the opportunity to undertake an internship at JDE’s R&D Centre in the UK during their candidature. The successful applicant will need to meet the criteria for admission, opens in a new window into the Higher Degree Research (Program Code: 1010) at UNSW and is expected to commence their studies by Term 3 of 2020.
Value
Full scholarship for up to 4 years, plus support for industry internship.
Closing date
30 June 2020
For further information, contact Prof. Cordelia Selomulya., opens in a new window
-
Supervisor
Prof Cordelia Selomulya | UNSW School of Chemical Engineering,
Prof Federico Harte | Department of Food Science, Penn State
Prof Xiao Dong Chen | UNSW School of Chemical and Environmental Engineering, Soochow University
Project summary
The Australian dairy industry plays a significant part in the nation’s economy, with almost $3 billion in export revenue in 2016-2017. Powdered dairy products extend shelf life and ease of transport, with >20% annual growth in premium products, such as milk protein concentrates and infant formula powders. This project aims to support the development of value-added dairy powders by investigating the impact of a novel processing technology in enhancing the properties of dairy powders and/or introducing new functionality. Successful outcomes will help expand the offering of high value dairy ingredients and thus increase the global competitiveness of Australian dairy manufacturing.
PhD scholarships
PhD scholarships are available for outstanding full-time postgraduate candidates to aid in the development of added-value dairy products by providing insight into the fundamental changes in surface compositions and properties of dairy powders in response to specific pre-treatment of feed emulsions.
One of the projects aims to correlate the changes in dairy emulsion properties under specific pre-treatment conditions (including high pressure jet) on powder functionality, by evaluating the modifications of protein structures, i.e. denaturation, aggregation, and interactions with fat droplets via dose response studies of pressure and protein/fat ratio. The knowledge gained will confirm the proposed hypothesis of the possible cause(s) of changes in functional properties for powders treated with different range of pressures, and potentially introduce a new functionality of the resulting powders.
Another project aims to develop a mechanistic understanding of surface formation on dairy powders by systematically monitoring the migration of solutes (fat, protein, lactose) of different emulsions and utilising the data to model the component distribution during drying. The understanding will contribute to the development of a more accurate model to predict surface-induced property changes of dairy powders.
Successful outcomes will help develop dairy powders with new / improved functionality, such as better encapsulation of fat or by introducing new surface functionality and desired properties relevant to applications (solubility, shelf stability, foamability) after rehydration. The applications can be extended beyond dairy to other food and nutraceutical products.
In addition to research work conducted at the School of Chemical Engineering, the PhD candidates will have the opportunity to conduct part of their research in our partner universities. Successful applicants will need to meet the criteria for admission, opens in a new window into the Higher Degree Research (Program Code: 1010) at UNSW and is expected to commence their studies in 2020 - 2021.
Value
Full scholarship for up to 4 years, plus support for research study exchange.
Closing Date for UNSW HDR admission, opens in a new window
18 September 2020
For further information, contact Prof. Cordelia Selomulya.
-
Supervisor
Associate Professor Jayashree Arcot | Food and Health Cluster | UNSW School of Chemical Engineering | Associate Professor Liang Qiao, Storr Liver Centre | Westmead Institute of Medical Research | University of Sydney
Project summary
In recent years, 2D human and animal intestinal cell cultures have been the main platform for studying the intestinal absorption of nutrients, and the nature of these studies is more reflective of bioaccessibility rather than bioavailability. The data from the existing approaches are of limited clinical implications. Therefore, more reliable methodologies are needed for studying bioavailability, a measurement of the amount of an ingested nutrient that is potentially available for absorption and transport.
This PhD project aims to develop a novel sequential model consisting of both human intestinal and liver cells for more physiologically and clinically relevant nutritional studies. With this novel 3D organoid model, we aim to simulate the human digestion using the Nutrascan gut digester, opens in a new window and sequentially study the bioaccessibility (nutrient uptake from the food sources) and bioavailability (transport and absorption) of micronutrients such as water soluble and fat -soluble vitamins and minerals from different food matrices.
Environment
This is a collaborative project and will be undertaken at the School of Chemical Engineering within Food and Health Cluster and at the Westmead Institute of Medical Research, Sydney.
-
Supervisor
Associate Professor Jayashree Arcot | Food and Health Cluster | School of Chemical Engineering, UNSW and Prof. Martina Stenzel | UNSW School of Chemistry
Project summary
Cellulose is the most abundant polymer on the planet, though it has only recently become popular in use as a plastic because of the development of novel separation techniques, which provides the cellulose with a more familiar plastic-like quality. Australia produces an estimated 3.7 billion bags or 21,000 tonnes of plastic that goes into landfills every year. These are plastics that will remain there virtually forever. The team in the last few years has developed a method of extracting nanocellulose from agricultural wastes and produced biodegradable films suitable as packaging material from banana pseudostems., opens in a new window This polymeric material from the waste is, opens in a new window composed almost entirely of cellulose, which is biodegradable. We are currently developing operations, methods, and technologies for cellulose producers to cheaply produce and market the starting material for biodegradable plastics, or furthermore, to completely produce plastics near the farm. This is something that is unique amongst traditional plastics. Some plastics can be transparent and soft, while others are white and hard. Some are great as water barriers while others excel at being impermeable to air for use in such areas as food packaging materials.
This PhD project aims to make the polymers with tailorable properties-with any characteristics that we choose from the same starting material, cellulose; customize the plastics that we produce to many targeted properties; develop ways to make plastics that are breathable or impermeable, absolutely water blocking or extremely hydrophobic, and having a range of strengths and stretchiness.
Environment
This is a project that will be undertaken in the School of Chemical Engineering.
-
Supervisor
Associate Professor Jayashree Arcot | Food and Health Cluster | UNSW School of Chemical Engineering
Project summary
The key contributors of bioactive compounds such as carotenoids (carotenes, xanthophylls), anthocyanins and several phenolic acids are several coloured vegetables and fruits. A few of them have also been shown to have preventative effects in pure forms such as lutein and Zeaxanthin on degeneration of the eye. A key property of these compounds are their anti-inflammatory activity and the positive impacts on prevention of some chronic conditions. While purified forms of these compounds have been studied widely, their presence in food and the matrix effects in terms of their bioavailability and also their bioactive properties have not been systematically studied which is also complicated by very little information on the composition of these compounds in foods. This project will first identify the best food sources of carotenoids; their interactions during digestion of foods and the best combinations of food sources to identify their bioactive effects (synergistic, antagonistic and enhancement) using 2D and 3D cell models.
Environment
This project will be undertaken in the School of Chemical Engineering.
-
Supervisors
Project summary
This industry PhD project will be conducted in partnership with Sanitarium Health Food Company, opens in a new window, the maker of iconic Australian brands including Weet-Bix™, Up&Go™ and So Good™, and the Future Food Systems CRC, opens in a new window.
The project aims to develop a quantitative understanding of parameters influencing the properties of wheat grains during drying, as an important step in the manufacturing process of Weet-Bix. The outcomes will help optimise Sanitarium’s drying operation and improve product quality by enabling better control of the drying process and, potentially, by improving the design of the dryers.
Key techniques
The project will suit a candidate with a strong background (H1 equivalent) in Chemical Engineering, Mechanical Engineering or related fields. The project will involve both practical and modelling components, and thus will suit a candidate with strong mathematical background, ability to conceptualise system design, and interest in a hands-on project related to food manufacturing.
All applicants need to meet the criteria for admission, opens in a new window into the Higher Degree Research (Program Code: 1010) at UNSW. Domestic candidates currently residing in Australia are preferred. The candidate chosen will be expected to commence their PhD studies by Term 1 of 2022. The successful candidate will receive an industry-funded scholarship via the Future Food Systems CRC. The scholarship provides an excellent opportunity for an outstanding full-time postgraduate candidate to undertake an industry-focused research project designed to solve a real-world challenge in food manufacturing.
Environment
In addition to research work conducted at the School of Chemical Engineering, the PhD candidate selected must be willing to travel between UNSW Sydney campus in Kensington and the Sanitarium R&D site at Cooranbong on NSW’s Central Coast to conduct experiments during their candidature.
Further information about this project can be found here. , opens in a new window
Value: A full scholarship of $35K per annum for up to four years, plus travel support and access to industry network and workshops as part of the Future Food Systems CRC.
Closing date for applications: 30 November 2021.
Polymer chemistry
-
Supervisor
Professor Per Zetterlund, opens in a new window, Email, opens in a new window | UNSW School of Chemical Engineering
CAMD research centre website, opens in a new window
Project summary
The material graphene was discovered in 2004 (Noble Prize awarded in 2010) – it is the strongest material ever measured, and this is accompanied by a range of other extraordinary physical properties such as high thermal conductivity and high electrical conductivity. Graphene is seen as the material of the future, with the potential to revolutionise a wide range of industries from electronics to healthcare, and there is currently immense worldwide research activity in this area.
The addition of graphene as a component of polymer nanocomposites can result in superior material properties – it is a way of combining “the best of both worlds” (polymer and graphene). However, both pristine graphene and graphene oxide are incompatible with most polymers, and do not form homogeneous polymer composites. This challenge can be overcome by use of aqueous emulsion polymerization techniques. In our group, we are designing and preparing novel polymeric nanocomposites and nanoparticles using graphene/graphene oxide and radical polymerization in environmentally friendly aqueous emulsion-based systems. Our research is leading to a range of novel materials and nanoparticles with potential applications in diverse fields such as the coatings industry and nanomedicine.
-
Supervisory team
Professor Per Zetterlund, Email, opens in a new window | UNSW School of Chemical Engineering
CAMD research centre website, opens in a new window
Project summary
Polymeric nanoparticles, i.e. particles with diameters around 100 nm comprising polymer(s), find applications in a wide range of areas, e.g. materials science (coatings) and nanomedicine (drug delivery). Future challenges in this area of research include synthesis of particles of specific composition/functionality as well as shape and size. In the present project, the aim will be to develop a novel method of polymeric nanoparticle synthesis by use of carbon dioxide. The cornerstone of the project is the generation of aqueous miniemulsions (organic droplets dispersed in a continuous aqueous phase) by use of compressed carbon dioxide. It entails the addition of carbon dioxide to a relatively low pressure of approximately 5 MPa to a stirred mixture of oil (e.g. vinyl monomer), water and surfactant, leading to formation of a miniemulsion without application of a high energy mixing device.
Under appropriate conditions, a transparent emulsion is formed, i.e. droplets with radius < 30 nm, and the particle size can be conveniently controlled by the carbon dioxide pressure. The process holds promise of great versatility, and has been successfully utilized with anionic, cationic and nonionic surfactants, but remains to reach its full potential with regards to synthesis of polymeric nanoparticles. Research Environment: The student will work in the well‐equipped Centre for Macromolecular Design (CAMD) laboratory alongside a large number of postgraduate students and postdoctoral researchers, the vast majority of whom are also involved in related research.
This will be an ideal and stimulating environment to learn what research is all about. Novelty and Contribution: Miniemulsion polymerization has long been heralded the technique of the future, but despite this still remains largely unexploited on the industrial level. The present project will lead to the development of low energy applications of the miniemulsion technology, with additional benefits in terms of easy adjustment of particle size via the carbon dioxide pressure. As such, the work is anticipated to be of high impact in the area of polymeric nanoparticle synthesis.
-
Supervisory team
Dr Jiangtao (Jason) Xu, Email | UNSW School of Chemical Engineering
Professor Cyrille Boyer, Email | UNSW School of Chemical Engineering
Project summary
This project aims to synthesise polymers that have precise chemical structure and mimic the biological activities of natural biopolymers like peptides and proteins. Monomer sequence and stereochemistry regulation in these natural biopolymers is important in biology and necessary for crucial features of life, such as molecular recognition, self-replication and catalysis. Current artificial techniques for biopolymer synthesis are time consuming and present low yields at high costs. This project expects its new materials will increase manufacturing sustainability, chemical diversity and industrial viability; produce health benefits for Australia by improving chemotherapy and diagnosis for diseases; and benefit the Australian economy.
-
Supervisory team
Dr Jiangtao (Jason) Xu, Email, opens in a new window | UNSW School of Chemical Engineering
Project summary
Bio-based polymers are attractive materials to address current global sustainability and to reduce the dependent of modern civilization on petrochemical industry. At present, most of the commercial monomers for industrial polymer manufacturing are petrochemical products which extremely rely on the unsustainable fossil fuel. Development of renewable monomers and their polymers is a high demand objective. This project aims to explore innovative green and sustainable technologies for transforming renewable biomass and abundant feedstocks from natural plants into high valued polymer materials. Meanwhile, this project also aims to harvest solar light by chemical means and utilize photo-energy as energy input for chemical reactions and renewable polymer manufacturing.
-
Supervisory team
Dr Jiangtao (Jason) Xu, Email, opens in a new window | UNSW School of Chemical Engineering
Project summary
Flexible and soft electronic devices have great potentials for various applications in bioelectronic skins, electrochemical supercapacitors, artificial intelligence systems, and human health monitoring. Considerable efforts have been recently devoted to fabricating electrically conductive hydrogels as advanced sensor devices due to their outstanding flexibility, stretchability and self-healing properties. Hydrogels are generally highly absorbent polymer networks that can accommodate large amount of water (more than 90%). With the availability of a broad range of environmentally benign natural or synthetic polymer materials for making the hydrogel networks, hydrogel-based sensor devices offer good biocompatibility for human skins.
-
Supervisor
Professor Cyrille Boyer, Email | UNSW School of Chemical Engineering
CAMD research website, opens in a new window
Project summary
In the past five years, light mediated polymerization has emerged as a powerful method for the preparation of functional polymers, due in part to its temporal and spatial control. In 2014, we developed a novel visible-light mediated polymerization technique, named photoinduced electron/energy transfer – reversible addition fragmentation chain transfer polymerization (PET-RAFT). In this project, we propose to use this technique for the synthesis of 3D functional materials, which will allow control over specific mechanical properties of these 3D objects. This project will result in the preparation of advanced materials with tuneable mechanical properties.
-
Supervisor
Professor Cyrille Boyer, Email | UNSW School of Chemical Engineering
CAMD research website, opens in a new window
Project summary
In the last ten years, new methods have emerged for the synthesis of functional polymers and their assembly into nanoparticles. These polymeric nanoparticles find a broad range of applications as nano-containers for the encapsulation of active and therapeutic compounds. However, the effect of nanoparticle shape has rarely been investigated. In this project, we propose the design of polymeric nanoparticles with precise control of size and shape using visible light. The effect of the size and shape of these polymeric nanoparticles will be studied for the delivery of therapeutic compounds in vitro. This project combines polymer synthesis and self-assembly processes with drug delivery applications
-
Supervisor
Professor Cyrille Boyer, Email | UNSW School of Chemical Engineering
CAMD research website, opens in a new window
Project summary
Antibiotic resistance is a growing threat for human health. Indeed, by 2050 an estimated 50 million people could be affected annually which will have a significant economic impact. In this project, we propose to design and investigate bioactive polymers capable of selectively killing bacteria. By synthesizing well-defined polymers, where each monomer unit is precisely placed in the polymer chain, we will correlate anti-microbial activities for a range of bacteria, with the polymer architectures and structures. To achieve this goal, we will use the most recent polymerization techniques, including photoinduced electron/energy transfer – reversible addition fragmentation chain transfer polymerization (developed by our group). This project combines polymer synthesis with microbiology and will be achieved in conjunction with international collaborators.
-
Supervisory team
Prof. Cyrille Boyer, Dr. Jin Zhang, Dr. Nathaniel Corrigan
https://www.boyerlab.com, opens in a new window
Project summary
Project details: This project aims to apply state-of-the-art living polymerisation techniques to 3D printing to efficiently produce customised polymer materials that are tailored at the molecular level. The effect of nanostructure on the macroscopic material properties will be investigated. The intended outcome of this project is to produce advanced materials with tailored mechanical properties via streamlined and accessible approaches. These new systems will produce highly structured polymer materials with remarkable mechanical properties. The interdisciplinary nature of the project, and diverse background of world class researchers working on this project, will provide the candidate with access to cutting edge equipment and training in new research areas such as advanced manufacturing and nanomedicine/biomaterials. This project will provide candidates with skills in nanomedicine, functional materials, and provide future proofed training for industrial and academic careers.
Location: Australian Centre of Nanomedicine School of Chemical Engineering UNSW Sydney, Kensington Campus
Stipend: $28,597 per annum
Pre-requisites/eligibility: The PhD Scholarship is available to students who have gained (or expect to gain), first class honours or equivalent, in relevant research areas. Recipients of this PhD Scholarship are required to obtain a Tuition Fee Scholarship (TFS, for international students), or be Australian citizens or permanent residents.
How to apply: Submit a Resume/CV and cover letter (as a single document) which includes:
- the reasons why the research project/s you have selected are of interest to you and how your skills/knowledge meet the project requirements;
- certified copies of your academic transcripts;
- the names and contact details of two academic referees;
Please submit all applications and questions to living3dprinting@gmail.com, opens in a new window
Applications close Sunday 31 January 2021
-
Supervisor
Dr Nicholas Bedford, Email, opens in a new window | UNSW School of Chemical Engineering
Associate Prof Jason Scott, Email, opens in a new window | UNSW School of Chemical Engineering
Project summary
The partial oxidation of methane is an intriguing route toward the production of syngas at stoichiometries directly amendable to methanol synthesis and/or Fischer-Tropsch reactions. This reaction is notoriously tricky to maintain, as it is often plagued with selectivity issues (i.e. total oxidation to CO2), or catalyst deactivation through coke formation. Additionally, the understanding of catalyst processing and structure is poorly understood, limiting logical decisions on catalyst design. This project aims to develop new materials with improved reactivity using single atom catalysts supported on silicon carbide/nitride supports made via block copolymer templating approaches. Preceramic polymers act as a precursor to form silicon carbide/nitride nanomaterials, while blending with appropriate catalyst metal precursors yields the final catalyst. To best understand how the materials are formed during processing and during partial oxidation of methane reactions, in-situ synchrotron characterization methods will be used to form structure/function relationships for these novel materials. The PhD project is funded through the American Chemical Society’s Petroleum Research Fund and will necessitate an array of skills including particle synthesis, materials characterization and catalytic performance assessment. The student will be expected to participate in the Annual ACS Spring Meeting in Years 2 and 3 of their PhD.
Key techniques
Block copolymer template formation, high temperature ceramic processing, gas phase thermal catalysis, materials characterization including transmission electron microscopy and synchrotron characterization methods such as X-ray absorption spectroscopy and high-energy X-ray diffraction.
Environment
The project will be undertaken within the Bedford Research Group, opens in a new window in the School of Chemical Engineering, which is part of the Particles and Catalysis Research Group., opens in a new window
For further information regarding this project, please contact Dr Nicholas Bedford, opens in a new window, Prof Jason Scott, or visit:
-
Supervisor
Dr Nicholas Bedford, Email, opens in a new window | UNSW School of Chemical Engineering
Project summary
Preceramic polymers provide unique ways to process otherwise cumbersome ceramic materials into interesting shapes and morphology at the nano, micro, and macro scale. The use of polymeric precursors further enables new chemistries and synthetic pathway not achievable using traditional ceramic processing. Yet the field is still in it infancy, and understanding how polymer chemistry and processing conditions influence the materials final structure and properties remains unknown. This project, in collaboration with the US Air Force Research Laboratory, aims to fill this void in fundamental knowledge by using a suite of advanced in-situ synchrotron structural characterization tools to understand how structure and polymer chemistry influences properties. The PhD project is funded through the Asian Office of Aerospace Development, where the student will be expected to participate in synchrotron experiments at the Advanced Photon Source and Cornell High-Energy Synchrotron Source in the US throughout the project.
Key techniques
Structural characterization of polymeric materials, the final ceramic nanocomposite, and the transitional structure in between is critical for the success of this project. As such, a prospective student should be eager to learn about various advanced characterization techniques, including X-ray absorption spectroscopy, small angle X-ray scattering, high-energy X-ray diffraction, and atomic force microscopy.
Environment
The project will be undertaken within the Bedford Research Group, opens in a new window in the School of Chemical Engineering, which is part of the Particles and Catalysis Research Group., opens in a new window
For further information please visit:
-
Supervisors
Professor Rita Henderson, opens in a new window, Email | AOM Lab, School of Chemical Engineering, UNSW
Dr Tony Granville, Email | SPARC Innovation Manager, Harrison SPARC
Dr Peter Wich, opens in a new window, Email | CAMD, School of Chemical Engineering, UNSW
Project summary
Metal coagulants and synthetic polymers, such as polyacrylamides, have been applied for decades to enhance separation in the water treatment and mining industries. However, these coagulants lead to the production of large sludge volumes, while synthetic polymers are derived from fossil fuels and are toxic. The development of novel, recyclable coagulants derived from naturally occurring materials is needed to improve the sustainability of separation processes. This PhD project will develop novel polymers and oligomers from natural-based polymeric backbones for applications in solid-liquid separation in the water and waste treatment industries. Polymer backbones will be modified as required for targeted applications, with a focus on the potential for surface charge and hydrophobicity design. The potential for polymer recycling will also be examined by designing responsivity to pH, temperature and light changes. These materials will be designed to enhance contaminant removal, lower dose rates, lower toxicity, and reduce fouling of downstream filtration.
Research environment
The project will be undertaken primarily in the UNSW School of Chemical Engineering, across both the Algae and Organic Matter Lab (AOM Lab, opens in a new window) and Centre for Advanced Macromolecular Design (CAMD). The candidate will also spend a significant proportion of time in the laboratories of Harrison SPARC, opens in a new window.
Candidate Requirements
The candidate should have a background in chemistry or chemical engineering (or similar), ensuring a solid background in chemical synthesis techniques, chemical characterisation, as well as a demonstrated aptitude for undertaking laboratory work. They should be able to demonstrate excellent communication skills, a creative and positive attitude to problem solving and an ability to work both autonomously and in a team. Industry experience while desirable, is not mandatory.
Process systems engineering
-
Supervisory team
Professor Jie Bao | School of Chemical Engineering, UNSW Engineering
Email: j.bao@unsw.edu.auProfessor Maria Skyllas-Kazacos, Professor Barry Welch | School of Chemical Engineering, UNSW Engineering
Project summary
The aluminium smelting process is very energy intensive. Australia’s smelting industry consuming 29.5 TWh of electricity in 2007, representing 13% of total electricity generated in Australia. The aluminium industry is facing significant challenges - variable availability of the electrical energy, particularly with increased penetration of renewable energy sources which are intermittent. Ideally, the production rate of the smelting process should be flexible through power modulation, optimized based on a number of factors including the aluminium price and energy availability/cost. However, the present approach to smelting cell control only allows limited flexibility for power modulation.
This project will develop new smelting process operation strategies, and cell monitoring and control approaches to allow flexible power modulation. There are major challenges in power modulation of aluminium reduction cells. Variable power input (amperage) may lead to significant problems in heat balance of the cells and current efficiency, and abnormal conditions may occur if the reduction cells are not tightly controlled.
This project is supported by the Australian Research Council (ARC) through its Research Hub for Integrated Solutions to Energy Storage and our industrial partner Emirates Global Aluminium (EGA).
The supervisory team includes Prof. Bao (an expert of process control with international repute), Prof. Skyllas-Kazacos (an international authority of electrochemistry) and Prof. Welch (an international authority of electrochemistry and aluminium smelting process).
-
Supervisory team
Professor Jie Bao | School of Chemical Engineering, UNSW Engineering
Email: j.bao@unsw.edu.auProfessor Wei Wang | School of Computer Science and Engineering, UNSW Engineering
Project summary
With implementation of digital control systems, a very large amount of process operation data is being collected in the process industry. This project will develop a novel data-centric control approach that utilizes the big process data to improve the cost-effectiveness of process operations. Based on the behavioural systems theory and dissipativity theory, integrated with machine learning techniques, this project aims to develop a novel big data-driven control approach for nonlinear chemical processes. Specifically, we will develop:
- A new approach to analyse, classify and organise a large amount of process operation data in behaviour trees for data-driven control, using the concept of system behaviours and machine learning techniques;
- A novel stability analysis and design for big data-driven control based on the dissipativity theory in the system behaviour context;
- A novel multi-time scale data predictive control approach which determines the control action using online input-output trajectory and information on process behaviours in the big process database to achieve optimal process economy and ensure system stability.
The outcomes are expected to benefit the Australian process industry, where many processes are controlled by inadequate logic controllers, by improving their operational efficiency.
-
Supervisory team
Professor Jie Bao, Email | School of Chemical Engineering, UNSW
Emeritus Professor Maria Skyllas-Kazacos | School of Chemical Engineering, UNSW
Professor Faz Rahman | School of Electrical Engineering and Telecommunications, UNSW
Professor John Fletcher | School of Electrical Engineering and Telecommunications, UNSW
Process Control research group website
Project summary
Distributed energy storage in electrical grids is becoming a critical aspect in maintaining power quality in the scenarios with a high penetration of renewable energy. The scope of this project includes:
Based on the dissipativity control theory, this research project aims to develop new scalable distributed control methods for a storage centric approach to distributed energy storage and power management, to improve power demand supply balance and achieve optimal techno-economic objectives including efficiency and reliability in both grids and standalone microgrids.
Online battery monitoring and optimal charging/discharging control approaches for Vanadium batteries will be developed by integrating battery design with control design.
Approaches will be developed to use Vanadium batteries for both power quality control and demand supply balance control, replacing the existing scheme that needs both supercapacitors for fast transient power control and batteries for slow dynamics.
This project is supported by a current ARC Discovery Projects grant.
-
Supervisory team
Professor Jie Bao, Email | School of Chemical Engineering, UNSW
Professor Sirish L. Shah | School of Chemical and Materials Engineering, University of Alberta, Canada
Process Control research group website
Project summary
Modern industrial processes are very complex, with distributed process units via a network of material and energy streams. Their operations increasingly depend on automatic control systems, which can make the plants susceptible to faults such as sensor/actuator failures. Occurrence of faults is increased by the common practice to operate processes close to their design constraints for economic considerations. This project will develop a new approach to detect and reduce the impact of these faults, which can cause significant economic, environment and safety problems.
Based on the concept of dissipative systems, this project aims to develop a novel integrated approach to distributed fault diagnosis and fault-tolerant control for plantwide processes. The key dynamic features of normal and abnormal processes are captured by their dissipativity properties, which are used to develop an efficient online fault diagnosis approach based on process input and output trajectories, without the use of state estimators or residual generators. Using the dissipativity framework, a distributed fault diagnosis approach will be developed to identify the locations and faults in a process network. A distributed fault tolerant control approach will be developed to ensure plantwide stability and performance.
This project is supported by a current ARC Discovery Projects grant.
-
Supervisory team
Professor Jie Bao, Email | School of Chemical Engineering, UNSW
Associate Professor Ian Manchester | School of Aerospace Mechanical and Mechatronic Engineering, University of Sydney
Dr Jinfeng Liu | School of Chemical and Materials Engineering, University of Alberta, Canada
Process Control research group website
Project summary
The process industry is continuously under the pressure from global competition and rising costs of energy and raw materials. With the globalisation of the world economy, the last decade has seen the increasing dynamics of the market demand with time varying diversity and quantity of products. This means the business in the process industry needs to have the flexibility to dynamically adjust the volume and specifications of the products and deal with diverse sources of raw materials and utilities to remain competitive. The process industry is at the dawn of next generation “smart manufacturing” (USA) or “Industry 4.0” (Germany) to transform towards demand-dynamic economics, performance based enterprises and demand-driven supply chain services by developing. Current research effort is focused on the development of the information technology architecture for the new generation smart plants. This project aims to develop a fundamental process control framework for this new industry paradigm.
Based on the behavioural approach to systems, dissipativity theory and contraction control theory, this project aims to integrate nonlinear control theory with distributed optimisation to develop a novel distributed economic predictive control approach for complex industrial processes that coordinates a network of autonomous controllers to control a plantwide process to achieve flexible targets (e.g., product specifications) according to market demand and maximises process economy. This outcome will be extended for distributed decision making for extended enterprises in global supply chain networks.
-
Supervisory team
Professor Jie Bao, Email | School of Chemical Engineering, UNSW
Emeritus Professor Maria Skyllas-Kazacos | School of Chemical Engineering, UNSW
Process Control research group website
Project summary
Primary production of aluminium is highly energy intensive, with energy costs representing 22-36% of operating costs in smelters. The long term sustainability of the aluminium smelting industry depends on energy-efficient production technologies for global competitiveness.
As an interdisciplinary project of process control and electrochemistry, this project aims to develop a new automatic control approach for aluminium smelting cells to provide much tight control of key distributed process variables in both spatially and temporally. The outcomes will be integrated in the control subroutines and work practices. This will significantly improve the energy and environmental efficiencies of operation of aluminium smelters.
This project is supported by one of the major aluminium companies.
-
Project summary
Coal represents one of the most important resources in Australian economy and coal research represents promising career opportunities in Australia. However, coal should be to more thermal-efficient and environmentally friendly fuel, i.e. clean oil and gas, for wider and cleaner applications. However, these processes are very complicated and challenging in design. In collaboration with coal industry, this project will study next generation coal upgrading technologies by combining numerical simulation and experiments.
Novelty and contribution
Using the advanced numerical modelling approach, the innovative process can be designed, illustrated and scaled-up in a cost-effective manner. For example, in our previous studies, a set of CFD models are developed to describe the Victoria brown coal pyrolysis from lab-scale to industrial-scale (right figure).
Expected outcome
This project will continue the effort in the model development for coal upgrading technologies as well as the understanding of the mechanism behind the complex thermochemical phenomena. A number of scientific articles and conference presentations will also be produced within the duration of the project.
We welcome talented students who are interested in the work. We have a variety of scholarships for domestic and international students.
-
Supervisor:
Professor Yansong Shen, ys.shen@unsw.edu.au, opens in a new window | UNSW School of Chemical Engineering | ProMO Website, opens in a new window
Project summary
Hydrogen is promising next generation renewable energy to reduce carbon footprint. Electrolysis is a promising route to produce hydrogen, however, this process has not been well design and optimised. This project aims to develop a high-efficient electrolysis system by combining CFD approach and lab experiment for exploring the optimised design, configuration and productivity.
Novelty and contribution
Supported by the Australian Renewable Energy Agency (ARENA), this project will develop new design tools for optimising the process of photo-voltaic electrolysis (PVE), in terms of e.g. bubbles resistance, ionic resistance, new membrane materials in the electrolyser. Subsequently, the optimisation can be applied to increase the electrolysis efficiency.
Expected outcome
This project will develop a comprehensive electrolyser model for simulating the complicated electrochemical phenomena in the electrolyser system and thereby providing the necessary information for the technology upgrading. A number of scientific articles and conference presentations will also be produced within the duration of the project.
The model development is challenging and demanding. We welcome students who have experience in water electrolysis process/electrolyser and CFD modelling, or are interested in solving challenging problems to join our group.
-
Supervisor
Professor Yansong Shen, ys.shen@unsw.edu.au | UNSW School of Chemical Engineering | ProMO Website
Project summary
Fluidized bed is one of the dominant chemical reactors for gas-solid mixing and chemical production, and have been widely used in both conventional and emerging industries, such as pharmaceutical, metallurgy, and mining, especially in Australia. By introducing a strong jet flow, the packed particles with large density, diameter or complex shapes in the fluidized beds are vigorously dragged and form a spouting channel with two circulation patterns. The spout deflection can be easily triggered by small disturbances, which means the spout channel alternatingly deflects in the lateral direction. This phenomenon not only deteriorates the flow uniformity and product quality but also limits the scale-up of the fluidized bed.
This project aims to carry out a comprehensive study on spout deflection via combining laser-based experimental method and particle-scale numerical method. The initial study has demonstrated that spout deflection has a higher gas-solid mixing efficiency than the existing flow pattern in applications. This program will continue to explore the application and control of spout deflection for further scale-up of fluidized beds.
We welcome talented students who are interested in this work. A variety of scholarships for domestic and international students is available. Besides, this research provides high-quality education that prepares you for your future career in various enterprises and research institutes.
-
Supervisor
Professor Yansong Shen, ys.shen@unsw.edu.au, opens in a new window | UNSW School of Chemical Engineering | ProMO Website, opens in a new window
Project summary
Australia is one of the driest inhabited continents, and wastewater treatment and purification are of great significance and importance in Australia. In the membrane-based purification process, the understanding of fines migration behaviors and fouling mechanisms based on particle scale can effectively help optimize the operation conditions and increase the purification efficiency.
In ProMO group, the state-of-the-art CFD-DEM (computational fluid dynamic-discrete element method) modelling approach will be further developed for understanding the insights on fines migration and removal across the membrane. Several projects are available in this area:
- Model development for describing the powder-solid-liquid behavior including powder, solid and liquid phases.
- The study of fundamental powder-solid-liquid behaviors including complex inter-phase interactions.
- The application of new numerical models on water treatment process, for example the microfiltration and granular filtration, and the analysis of fouling mechanism based on particle scale.
Talented students who are interested in these projects are most welcomed, and various scholarships are available for both domestic and international students. The research experience during PhD study will prepare you for job prospects in research institutions or universities.
- Model development for describing the powder-solid-liquid behavior including powder, solid and liquid phases.
-
Supervisor
Professor Yansong Shen, ys.shen@unsw.edu.au | UNSW School of Chemical Engineering | ProMO Website
Project summary
As carbon neutral fuel, biomass has been reported one of most feasible and low-cost renewable energy source for future energy supply, representing 14% of the total amount of renewable energy at present. Biomass gasification is an innovative technology for converting diverse types of biomass into clean synthesis gas, transportation fuels, chemicals, and other products. This project aims to develop next generation biomass upgrading technologies towards to increased efficiency, low cost, and low greenhouse gas emission. Several PhD topics are available at ProMO group.
- Biomass gasification including model development, new process design, mechanism study, and industrial application.
- Biomass combustion in ironmaking blast furnace including model development, sub-model development and operational optimization.
- Co-gasification or co-combustion of biomass and other fuels, including used tire and coal.
We welcome talented students who are interested in the work, and we have a variety of scholarships for domestic and international students.
-
Supervisor
Professor Yansong Shen, ys.shen@unsw.edu.au | UNSW School of Chemical Engineering | ProMO Website
Project summary
Ironmaking blast furnaces is the dominant reactor to convert iron ore to iron products. The design and control of ironmaking BFs must be optimized to be competitive and sustainable, particularly under the more and more demanding economic and environmental conditions.
This project aims to study in-furnace multiphase flow, heat and mass transfer, and performance of ironmaking BFs, toward achieving reliable, cost- and energy-effective, and low-emission production ultimately. The discrete-based modeling methods with particle-scale information captured and the continuum-based modeling methods with macro-scale information obtained are developed in our ProMO group to efficiently unveil the features of locale and global multiphase flow and thermochemical behaviors inside BFs.
We welcome talented students who are interested in the work, and we have a variety of scholarships for domestic and international students. The research experience and skills acquired during PhD study will help developing high end job opportunities in global leading iron ore companies.
-
Supervisor
Professor Yansong Shen, ys.shen@unsw.edu.au | UNSW School of Chemical Engineering | ProMO Website
Project summary
Sand filtration is commonly used in drinking water treatment processes to remove suspended solids, colloids, and some pathogens like microbes and viruses. To improve the sand filtration performance, understanding the mechanics of hydrodynamics and adhesive torques between the suspension and sand particles is essential. This project aims to predict the adsorption or detachment of colloids and quantify the flow velocity heterogeneity near the sand particles surface. The Lattice Boltzmann method (LBM), which describe fluid transport phenomena by a resolved computational fluid dynamics (CFD) method, can describe the fluid-particle interactions. This project will focus on the LBM development for describing the fluid flow through porous media, aiming to deliver the fundamental understanding of liquid-solid hydrodynamics at particle scale and expand the application of sand filtration in drinking water treatment processes.
We welcome talented students who are interested in the work, and we have a variety of scholarships for domestic and international students. The research experience and skills acquired during PhD study will help exploring job opportunities in academia and industry.
-
Supervisor
Professor Yansong Shen, ys.shen@unsw.edu.au | UNSW School of Chemical Engineering | ProMO Website
Project summary
Particle mixing is an essential process to control product quality produced from particle blends in many industries, such as pharmaceutical, food and chemical engineering industries. Hence, the predictability and optimization of the mixing behavior of granular materials play a crucial role in energy-saving in these industrial scenes. This project aims to develop an advanced numerical technique - discrete element method (DEM), and to investigate particle mixing performance in different mixers (e.g., cylindrical mixer, ribbon mixer and rotating drum) for the prediction of the mixture quality.
Research environment
The PhD student will work in ProMO group and be part of Future Food System CRC centre. We will provide various domestic and international scholarships with talented students who are interested in process modelling.
Expected outcome
It is expected that the successful candidate will participate in international conferences and will publish his/her work in high impact factor journals.
-
Supervisor
Professor Yansong Shen, ys.shen@unsw.edu.au | UNSW School of Chemical Engineering | ProMO Website
Project summary
Coal remains the most widely used energy resource in the foreseeable future. Chemical looping combustion (CLC), which has intrinsic merit of separating CO2 during the combustion process, has been regarded as one of the most promising clean coal combustion technologies with near-zero emission of pollutants. However, the multi-scale structures and multi-physics processes of multi-phase flow in the fuel reactor (FR) and air reactor (AR) of the CLC require an in-depth understanding for the further design and optimization of industrial-scales apparatus.
This project aims to design next generation CLC system via a series of numerical studies of CLC systems using advanced numerical modelling approaches. Previously, the two-fluid model combined with thermochemical sub-models was developed to study the physical-thermal-chemical characteristics of dense gas-solid reaction flows in a CLC system. This project will continue to explore the application and optimization of the CLC process in aspects of reactor design, oxygen carrier selection, and reaction kinetics simplification.
We welcome talented students who are interested in the work, and we have a variety of scholarships for domestic and international students. The research experience and skills acquired during PhD study will help explore job opportunities in this promising area.
-
Supervisor
Professor Yansong Shen, ys.shen@unsw.edu.au | UNSW School of Chemical Engineering | ProMO Website
Project summary
One important topic in Industry 4.0 trend is smart manufacturing in conventional and emerging industries. This project aims to develop an innovative data-driven modelling approach for online describing complex multiphase flow and real time prediction of operational anomalies. At this point, ProMO group handles complex nonlinear problems using state of the art numerical methods including artificial neural networks (ANN), support vector machine (SVM), and the random forest (RF).
Novelty and contribution
This is a promising new research area at the frontier of applying novel data-driven method in chemical engineering fields. It is part of the search of alternatives to traditional computational fluid dynamics (CFD) processing technologies to achieve high-efficiency prediction of chemical reactors, therefore, enhancing reaction performance.
Expected outcome
It is expected that the successful candidate will participate in international conferences and will publish his/her work in high impact factor journals. This work will expand the capabilities of the student in both industrial and academic career.
-
Supervisor
Professor Yansong Shen, ys.shen@unsw.edu.au | UNSW School of Chemical Engineering | ProMO Website
Project summary
The current renewable devices fail to achieve satisfactory efficiency, due to lacking the in-depth understanding of the materials processing and materials properties. The phase diagram is a powerful tool to characterize and select potential materials. This project will develop phase diagrams for renewable energy-focused materials by combing the experimental methods and thermodynamic optimization methods using XRD, SEM-EDS, DTA, and CALPHAD technique. This project will contribute a set of reliable thermodynamic database of the related renewable energy materials and also work as a cost-effective tool to design scientific experiments and manufacture of alloy.
We consumingly welcome the further PhD graduates and will provide various scholarships for domestic and international students. Moreover, the research experience and skills acquired during PhD study will secure job opportunities.
-
Supervisor
Professor Yansong Shen, ys.shen@unsw.edu.au | UNSW School of Chemical Engineering | ProMO Website
Project summary
Biomass gasification is a thermochemical conversion process where the solid biomaterial are converted to combustible gases with minimal harmful emissions. This project aims to explore the hydrodynamics and thermochemical characteristics of dense gas-solid reaction flow during the biomass process by means of a CFD-DEM coupling approach. To capture these characteristics, the model should fully consider the heat and mass transfer sub-model, and the homogeneous and heterogeneous chemical reactions sub-models. This project will unveil the multi-scale flow structures and multi-physics phenomena involved in the biomass gasification process, benefiting the design and optimization of the relevant reactors.
The talented students with enthusiasm in coding and programming are welcome, and our group will provide a variety of domestic and international scholarships. It is expected that the successful candidate will participate in international conferences and will publish his/her work in high impact factor journals. This work will expand the capabilities of the student in both the industrial and academic career.
-
Supervisor
Professor Yansong Shen, ys.shen@unsw.edu.au | UNSW School of Chemical Engineering | ProMO Website
Project summary
Plastic recycling is an important issue with the shortage of the landfill and environmental pollutions as well as its economic impact. Residual adhesives should be removed for 100% HDPE recycling in massive plastic bottles recycling. It is necessary to design a process at laboratory and upscale the process from laboratory scale to industrial scale. To achieve this goal, an advanced mathematical model will be developed for describing the complex multiphase flow during the removal process and understanding the details of internal phenomena inside the reactor. A laboratory test rig will be set up for concept proof and model validation. Then, the mathematical model will be used to evaluate and test new designs and different operational scenarios extensively. In the end, the optimal design and process parameters combination can be obtained. Mathematical modelling, supported by laboratory-scale experiments offers a powerful and cost-effective tool for process design and optimization, and more importantly for industrial upscaling, from laboratory-scale concept proof to industry scale technology demonstration and implementation.
We welcome students to join us, using the advanced numerical modelling technology to protect the environment and make contributions to society. We provide various scholarships for international and domestic students. You can obtain good opportunities to participate in the collaboration between university and industry.
-
Supervisor
Professor Yansong Shen, ys.shen@unsw.edu.au | UNSW School of Chemical Engineering | ProMO Website
Project summary
Photovoltaic (PV) is one of the renewable technologies and the amount of waste PV panel is estimated to reach 9.57 million tonnes in 2050. The recycling of waste PV panels represents a challenge and also a business opportunity. This project aims to develop and design an innovative process of recycling end-of-life solar cell panels by means of numerical simulations for process design and lab experiments for process demonstration. The recycling sub processes will be designed and optimised in terms of EVA separation, hydrometallurgy, waste gas and water treatment, etc. From the engineering point of view, each process requires deep understanding so that the process optimisation can be achieved. Based on the proposed recycling technical scenario, the respective numerical model of each process will be developed to illustrate the physics and thermochemical phenomena behind it. A number of scientific articles and conference presentations will also be produced within the duration of the project.
We welcome the students who are interested in PV and numerical modelling technology to join us, and we could provide various scholarships for international and domestic students. You can obtain good opportunities to participate in the collaboration between university and industry.
-
Supervisor
Professor Yansong Shen, ys.shen@unsw.edu.au | UNSW School of Chemical Engineering | ProMO Website
Project summary
Hydrogen is the most promising energy source that can be applied environmentally friendly and economically. One key issue in hydrogen industry is to effectively store hydrogen. This project aims to conduct the process and design optimisation of the hydrogen storage system, including the internal structure configuration, materials, and operating conditions. For improving the performance of the storage system, a series of new designs will be proposed and numerically examined to evaluate their performance. It is expected that we can achieve the technological innovation of hydrogen storage in the near future. A number of scientific articles and conference presentations will also be produced within the duration of the project.
We welcome the students who are interested in renewable energy and numerical modelling technology to joins us, and we could provide various scholarships for international and domestic students.
-
Supervisor
Professor Yansong Shen, ys.shen@unsw.edu.au | UNSW School of Chemical Engineering | ProMO Website
Project summary
The migration of fine particles in gap-graded soils due to seepage flow is often encountered in geotechnical engineering. The mechanism of the seepage-induced fine particle migration is not yet clear since it involves the fluid flow through a complex pore network of the coarse skeleton at the pore-scale and the particle-fluid interactions at the particle-scale. This project aims to develop a semi-resolved CFD-DEM model which combines resolved CFD-DEM with the unresolved CFD-DEM to provide a useful tool in understanding the mechanism of fine particle migration. The fluid flow in the vicinity of the fine particles is locally averaged over a domain with dimensions larger than the sizes of fine particles, which is similar to that in the unresolved CFD-DEM; while the flow around the coarse particle is resolved using fluid meshes with sizes several times smaller than the diameters of the coarse particles, which is identical to that in the resolved CFD-DEM.
We welcome the students who are interested in computer science and numerical algorithm implementation on the opensource software packages to joins us, and we could provide various scholarships for international and domestic students
Environmental Processes and Technologies
-
Supervisor
Associate Professor Pierre Le-Clech, Email | School of Chemical Engineering, UNSW
UNESCO Centre for Membrane Science and Technology website, opens in a new window
Project summary
Given the potential risk associated with Legionella growth and persistence in engineered processes such as aerator systems used during water treatment, this project will be commissioned to describe our current understanding of aeration processes and their operating challenges, especially in a temperate climate. The identified gaps in knowledge are to be used to develop the following research activities:
- Improved risk assessment through quantitative measurement of aeration performance,
- Investigation of appropriate options for mitigation, cleaning and maintenance of current aerators,
- Development of guidelines for new aerator design,
- Development and assessment of appropriate monitoring and modeling tools for process performance and maintenance.
Research environment
Not only the student will be working within the world renowned UNESCO Centre for Membrane Science and Technology, this project will be based on significant collaboration with our industrial partner, Water Corporation in Western Australia. Given the nature of the study, many aspects will rely on complementary skills offered by the research team, at both UNSW and Water Corp. This will offer a great opportunity for the student to significantly increase his/her core knowledge within a wide range of topics.
Novelty and contribution
The practical contribution of this project on the health and safety of the plant operators and local community is of great significance. The access to plants combined with advanced sampling and analysis of aerators deposits will offer new insights to this challenge, and the development of new operational strategies. In addition, new analytical tools and statistical tests will be developed for this specific application.
Expected outcome
Ultimately, the project is expected to produce a set of guidelines to be used within the water industry for the design, operation and maintenance of aerator systems in water treatment. Obviously, a number of scientific articles and conference presentations will also be produced within the duration of the project.
-
Supervisor
Dr Francisco Trujillo, Email | School of Chemical Engineering, UNSW
Project summary
Ultrasonic Assisted Plasma Detoxification (UAPD) is a novel process for complete mineralization of recalcitrant pollutants in wastewater. Ultrasound is injected into the fluid via a horn that emits acoustic waves also producing cavitation bubbles in the liquid. This is because high-power ultrasound results in the expansion, compression and final implosion of gas micro-sized bubble dissolved in the liquid phase. The extreme collapse of the bubbles produce local temperatures of up to 5,000oK and pressures of up to 50 MPa. On the other hand, underwater plasma is produced via Radio Frequency electromagnetic waves. The mechanism of this technology is still unknown but is based on the synergism of cavitation bubbles that facilitate and sustain the plasma formation. The plasma produces radical species that mineralize pollutants. The advantage of this new technology is that it can completely detoxify and sanitize wastewater while producing hydrogen that can be used as a clean source of energy.
Research environment
The PhD student will have the opportunity to work with members of the UNESCO Centre for Membrane Science and Technology as well as wastewater treatment groups within the school of chemical engineering.
Novelty and contribution
Developing a new technology that potentially can achieve complete water detoxification and sanitation while producing hydrogen.
Expected outcome
It is expected that the successful candidate will participate in international conferences and will publish his/her work in high impact factor journals. This work will expand the capabilities of the student to work in the industry or to continue a research or academic career.
-
Supervisor
Dr Rita Henderson, Email, opens in a new window | School of Chemical Engineering, UNSW
Project summary
Surface water organic matter is frequently subject to characterisation using techniques such as size exclusion chromatography with trace organic carbon detection and fluorescence excitation-emission spectroscopy. However, there is a distinct lack of understanding as to the molecular composition of the size fractions of fluorescent peaks that are observed. This project seeks to apply advanced molecular characterisation techniques, for example NMR and high resolution mass spectrometry, and data analysis techniques to elucidate the composition of chemical structures.
-
Supervisor
Associate Professor Rita Henderson, Email | School of Chemical Engineering, UNSW www.aom.unsw.edu.au, opens in a new window
Project summary
Algal blooms in catchments and water reservoirs can produce nuisance and harmful metabolites that are carefully monitored and treated to maintain a high-quality water supply. However, algal growth and accumulation has also been observed within drinking water treatment plants (DWTPs). Some of these algae evidently penetrate from water reservoirs into the DWTP, and it is likely that DWTPs act as incubators for further algal growth. It is hypothesised that the DWTP ecosystem selects for, and incubates, particularly resilient algal species. This project will use state-of-the-art technologies to detect, quantify and characterise algal blooms in DWTPs, including genetic tools to investigate algal community composition and trace organic analysis to measure undesirable algal metabolites. To penetrate the DWTP, algae need to survive various physical and chemical water pre-treatment processes, which may include pre-oxidation, chemical coagulation-flocculation and rapid mixing. This project will therefore also investigate the impact of treatment processes on the composition of these resilient algal communities.
Research environment
The project will be undertaken in the Algae and Organic Matter Lab (AOM Lab) in the School of Chemical Engineering which is part of the world renowned UNESCO Center for Membrane Science and Technology ( www.membrane.unsw.edu.au, opens in a new window). The project falls under the ambit of the larger Nuisance and Harmful Algae Science-Practice Partnership (NHASP) with Melbourne Water ( http://www.algae.unsw.edu.au/, opens in a new window), which is a multi-party initiative that seeks to more effectively manage algal blooms by introducing smart surveillance and evidence-based, cost-effective policy and asset design for the benefit of the Melbourne region and Australia. For further information about AOM Lab, please visit: www.aom.unsw.edu.au., opens in a new window
Candidate requirements
The successful candidate(s) will join the NHASP program. The candidate should have a background in either civil, chemical or environmental engineering (or similar), a demonstrated aptitude for undertaking laboratory/field work, have excellent communication skills and will be expected to interact regularly with industry partners.
-
Supervisor
Associate Professor Pierre Le-Clech, Email | School of Chemical Engineering, UNSW
UNESCO Centre for Membrane Science and Technology, opens in a new window
Project summary
The filter backwash recycling rule (FBRR) has been introduced by the US EPA in 2002, and has been since implemented around the world as best practice to address the risk associated with pathogens present in supernatant waters (i.e., spent filter backwash water, sludge thickener supernatant and liquids from dewatering processes). Since its introduction, the FBRR has been considered as a significant factor limiting the opportunity to improve practices for supernatant return in drinking water plants. As increasing water scarcity in Australia forces utilities to continuously optimise their separation systems and their water recovery, it is now required to critically demonstrate the health risk associated with supernatant return and establish recommendations for new set of guidelines. Based on the critical importance of water quality and customer health protection, recent advances in risk assessment techniques are expected to allow the development of improved, more appropriate, but still safe management of supernatant return.
Research environment
Not only the student will be working within the UNESCO Centre for Membrane Science and Technology,, opens in a new window this project will be based on significant collaboration with a large number of industrial partners, especially water utilities like TasWater, Seqwater, Hunter Water… Given the nature of the study, many aspects will rely on complementary skills offered by the research team, from process design, operating management and control to microbial risk assessment. This will offer a great opportunity for the student to significantly increase their core knowledge within a wide range of topics.
Novelty and contribution
The practical contribution of this project on the health and safety of water consumers is of great significance. The access to plants combined with advanced sampling and analysis of supernatant treated by a range of processes will offer new insights to this challenge, and the development of new operational strategies. In addition, new analytical tools and statistical tests will be developed for this specific application.
Expected outcome
Ultimately, the project is expected to produce a set of guidelines to be used within the water industry for the design, operation and control of best supernatant recycling scheme in water treatment. Obviously, a number of scientific articles and conference presentations will also be produced within the duration of the project.
-
Supervisors
Professor Rita Henderson, opens in a new window, Email | AOM Lab, School of Chemical Engineering, UNSW
Dr Tony Granville, Email | SPARC Innovation Manager, Harrison SPARC
Dr Peter Wich, opens in a new window, Email | CAMD, School of Chemical Engineering, UNSW
Project summary
Metal coagulants and synthetic polymers, such as polyacrylamides, have been applied for decades to enhance separation in the water treatment and mining industries. However, these coagulants lead to the production of large sludge volumes, while synthetic polymers are derived from fossil fuels and are toxic. The development of novel, recyclable coagulants derived from naturally occurring materials is needed to improve the sustainability of separation processes. This PhD project will develop novel polymers and oligomers from natural-based polymeric backbones for applications in solid-liquid separation in the water and waste treatment industries. Polymer backbones will be modified as required for targeted applications, with a focus on the potential for surface charge and hydrophobicity design. The potential for polymer recycling will also be examined by designing responsivity to pH, temperature and light changes. These materials will be designed to enhance contaminant removal, lower dose rates, lower toxicity, and reduce fouling of downstream filtration.
Research environment
The project will be undertaken primarily in the UNSW School of Chemical Engineering, across both the Algae and Organic Matter Lab (AOM Lab, opens in a new window) and Centre for Advanced Macromolecular Design (CAMD). The candidate will also spend a significant proportion of time in the laboratories of Harrison SPARC, opens in a new window.
Candidate Requirements
The candidate should have a background in chemistry or chemical engineering (or similar), ensuring a solid background in chemical synthesis techniques, chemical characterisation, as well as a demonstrated aptitude for undertaking laboratory work. They should be able to demonstrate excellent communication skills, a creative and positive attitude to problem solving and an ability to work both autonomously and in a team. Industry experience while desirable, is not mandatory.
-
Supervisors
Professor Rita Henderson, opens in a new window, Email | AOM Lab, School of Chemical Engineering, UNSW
Dr Helen Rutlidge, opens in a new window, Email | AOM Lab, School of Chemical Engineering, UNSW
Project summary
Natural organic matter (NOM) is a complex mixture of a wide range of compounds. There is growing evidence that changes in the molecular composition of NOM can influence the effectiveness of water treatment processes. High resolution mass spectrometry is one such technique that is being used to provide insights into the molecular composition of NOM. However, due to its high sensitivity instrumental performance variance from day to day is a recognised problem, which impacts on the ability to compare samples from different time periods as well as understand the temporal changes in molecular composition of NOM. This project will investigate the impact of the instrumental variation and ways to overcome it. This will include determining an appropriate internal standard and how this minimises the influence of the instrumental variation. In addition, different data analysis approaches will be explored.
Research environment
This project will be based in the AOM Lab research group, opens in a new window in the UNSW School of Chemical Engineering. The candidate will work closely with technical staff in the Bioanalytical Mass Spectrometry Facility, opens in a new window (BMSF) part of the Mark Wainwright Analytical Centre at UNSW.
Candidate Requirements
The candidate should have a background in chemistry or chemical engineering, with a strong interest/foundation in organic matter and chemical composition. They should also have a demonstrated aptitude for undertaking laboratory work, data handling and data analysis.