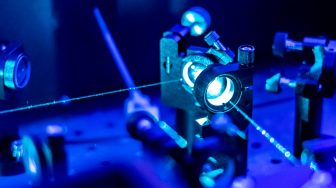
You can challenge yourself as an undergraduate physics student by working on a cutting-edge research project with our world-class researchers.
In your first year, the ‘Step into Research’ program offers the chance to undertake a small research project with one of the research groups in the school. In second and third years, it’s through the 'Taste of Research' program.
In first year, research projects are done on a voluntary basis, and in higher years there is the option of course credit. If you are applying for credit you must formally enroll in PHYS4200, SCIF2041, or SCIF3041. You can read more about these below.
First year
First year
All first year research projects are done on a voluntary basis.
Second & third years
PHYS4200
Physics Research Project
SCIF2041
Research Internship A
SCIF3041
Research Internship B
Discover our research projects
Now that you’re familiar with course structures, explore the research projects you can work on through the 'Taste of Research' or 'Step into Research' programs. Below our researchers share the details of projects they are leading. These projects are available to undergraduate students in 2024.
-
Looking back in time to the early Universe
Our group uses some of the world’s largest radio telescopes to measure some of the Universe’s faintest signals. We study the Epoch of Reionization, the time period when the very first stars and galaxies were born. Since the signal is so faint, petabytes of pristine data are required to measure the signal; while there are many telescopes around the world trying to measure this signal, no one has ever seen it. Potential projects would include trying to ascertain the contamination in the measured data to reveal the underlying signal through python programming, machine learning, and statistical representations. Skills obtained through this project would be useful for both astrophysics and data science career paths. -
We investigate new materials, devices and qubits with a focus on systems that may have applications in a future quantum computer made from spins, superconductors or topological systems. Experimental projects may involve activities ranging from device design and fabrication in a clean room environment, electromagnetic simulation, cryogenic measurement in dilution refrigerators, as well as developing control and readout software for qubit measurement. Please contact me to discuss a project topic that aligns with your interests and skills.
-
Quantum computing
Quantum computation focuses on using quantum bits to encode information. Unlike classical bits, which can be either 0 or 1, quantum bits exploit the superposition principle and can be in any combination of 0 and 1, which can make computation considerably faster and open new avenues that are inaccessible with classical bits. The two key problems facing the community at present are increasing the lifetimes of quantum bits (coherence), which determines how long quantum information can be stored, and devising ways to couple two or more bits so that complex operations can be performed in practice (entanglement). The research projects will focus on these two phenomena, devising novel strategies to beat decoherence mechanisms and to control interactions between quantum bits.
Topological quantum matter
In recent years a large number of physical phenomena have been ascribed to topological mechanisms, in which the curvature of the eigenspace of the system plays a vital role in determining the robust quantisation of response functions. These phenomena are so widespread nowadays that the 2016 Nobel Prize was awarded to three scientists who revealed their topological nature, and the Australian Research Council has established the Centre of Excellence in Future Low-Energy Electronics Technologies to investigate topological materials and effects. The research projects will focus on establishing the role of topological terms in the response functions of a series of newly discovered materials, including topological insulators, Weyl semimetals, and transition metal dichalcogenides.
-
Research interests of our group include:
- Atomic and nuclear theory and high precision atomic calculations
- Properties of superheavy elements
- Atomic and nuclear clocks
- Violation of the fundamental symmetries and tests of Grand Unification theories
- Dark matter models and search for dark matter
- Search for cosmological variation of fundamental constants in an evolving Universe
- Quantum chaos and statistical theory of small systems
- Quantum effects in strong gravitational field and black holes
-
My group’s research revolves around the physics of the early Universe and in particular what observations can tell us about the Universe’s history, composition and origin. Depending on students' prior knowledge and interest, I offer projects on topics such as cosmic inflation, Big Bang Nucleosynthesis, the cosmic microwave background, the formation of the Universe’s large scale structure, or machine learning for data analysis.
-
I am offering research projects in experimental condensed matter physics, studying the electronic properties of advanced quantum devices and topological materials. Potential projects include
- Spin-3/2 qubits in silicon quantum dots
Electrons are spin ½ particles whereas positively charged holes are spin-3/2, and make very fast quantum bits. We study extremely fast qubits in silicon quantum devices. - Measuring the quantum Hall effect in two-dimensional systems
The quantum Hall effect has been the subject of 3 separate Nobel prizes. Here you will measure the quantum Hall effect in 2D systems at temperatures below 2 Kelvin and magnetic fields up to 9 Tesla.
- Studying quantum electrical transport in one-dimensional nanowires
- Putting the flow back into electrical currents - hydrodynamics of electrons in quantum devices
We often talk of "electrical currents"; here we are exploring the viscous nature of the electrical fluid that flows in transistors.
- Making and measuring atomically thin transistors using graphene and the "sticky tape" fabrication approach
These projects will be rather "hands-on", with students working with researchers in our group to perform experiments themselves, taking their own data, and in some cases handling liquid cryogens and helium refrigerators.
- Spin-3/2 qubits in silicon quantum dots
-
My research group works in the area of Galactic archaeology, using the present-day properties of the Milky Way to investigate its history and evolution. I am offering projects studying the chemical compositions and orbits of stars in our galaxy, including:
- Identifying stars that have been captured from other galaxies
- Data mining in large survey data sets to find stars with anomalous compositions
- Determining the orbits and initial properties of stellar streams in the Galactic halo
These projects all involve python programming, and no prior programming experience is required.
-
Coherent spin manipulation and control of organic electronics
Coherent quantum effects are usually associated with extremely low temperature, making widespread technological applications difficult. Projects in the Centre for Exciton Science will investigate organic semiconductors, a class of material which exhibit quantum coherence in devices operating at room temperature. Projects may involve activities ranging from device design and fabrication in an oxygen free environment, to developing experimental systems for room temperature coherent control of spin states. The goal is to perform electrically or optically detected spin resonance experiments on a range of organic molecules, thereby increasing our understanding of spin coherence in these materials. Students interested in this area are encouraged to contact Dane to design a project that fits their interest and skills.
Spin based spectroscopy of light harvesting and modifying materials
The interaction of light with molecules leads to the formation of excitons. Projects in the Centre for Exciton Science will investigate molecular and nanoscale systems that allow engineering of the light spectrum via a range of spin dependent exciton interactions. Projects may involve activities ranging from device design and fabrication in an oxygen free environment, to developing experimental systems for optically exciting and measuring the influence of spin in these novel materials. The goal is to increase our understanding of electronic processes which can improve the efficient conversion of light into charge, and therefore improve energy generation in photovoltaic materials. Students interested in this area are encouraged to contact Dane to design a project that fits their interest and skills.
-
My research group studies exoplanets--worlds orbiting stars other than our own sun--and the physics of their host stars. To answer these questions, we use data from the NASA Kepler and TESS missions, two surveys that together have obtained long-term observations of the brightnesses of millions of stars. These data can be used to detect the presence of planetary systems as well as understand stellar activity like starspots, flares, and magnetic cycles. Students involved in these projects will have the opportunity to work with large data sets and explore statistical data analysis methods to study planets, stars, and interactions between the two.
-
My group’s research involves using the latest theoretical and computational techniques to describe living matter--- from cells to tissues to organisms. The overarching goal is to extend the remit of classical physics, and push biology and medical science towards working hand-in-glove with theory and computation, much like present day engineering. We are based in the Lowy Centre for Cancer Research and work alongside experimental partners who operate at the cutting edge of modern biology and microscopy. Students involved in this work will gain exposure to subjects such as genetics, molecular biology, mechanosensation, virology, and cancer research, as well as theory and computer simulation / numerics.
-
My research involves the theory and simulation of quantum materials and devices. My group has developed a number of computational tools and techniques to model and simulate physical mechanisms governing qubit operations, lifetimes, and coherence. With these methods, we collaborate with experimental groups to understand measurements, and to design and optimize quantum hardware. Our specialization is on silicon qubits hosted in dopants and quantum dots, but we also work on III-V material qubits. We also work on nano-electronic devices, including novel transistors beyond Moore's Law. Our goal is to utilize novel properties of emerging materials such as 2D and topological materials to design novel transistors and optical devices.
-
Manipulating nanoparticles with holographic optical tweezers
Optical tweezers use the momentum carrying properties of light to confine and manipulate nanometre- and micrometre-scaled objects at the focus of a high magnification microscope objective lens. Combining optical tweezers with holographic techniques allows for the direct manipulation of multiple trapped objects in three dimensions. In this project you will create digital holograms for the purpose of manipulating nanoparticles in amusing ways. Along the way you will (hopefully) learn about optical tweezers, adaptive optics, Fourier optics, holography and nanoparticle physics.
-
My group works on coupling qubits in a robust manner from the atomic nano-meter length scale to macroscopic coupling via photons. On the atomic scale we optimise the coupling between donor atoms while taking the complex nature of their charge density in silicon into account. To achieve macroscopic coupling we engineer electric-dipole coupling to spin qubits. This enables direct qubit-qubit coupling at the 100nm length scale and the coupling to microwave resonators that allows for chip scale coupling. For the optical interface to spin qubits we establish long distance coupling based on rare-earth ions in silicon with compact optics and integrated single-photon detectors in collaboration with ANU, Griffith, RMIT, and UNSW Canberra.
-
Quantum simulation using donor-based quantum dots
Phosphorus-doped silicon quantum dots fabricated with scanning tunnelling microscopy lithography show promise for analogue quantum simulation. We will be performing research on potential applications of these devices for analogue quantum simulation of various physical systems.
Fast, high-fidelity spin readout
Electron spin readout requires the application of fast voltages to control the potential of quantum dots. We will be examining the use of machine learning to optimise the accuracy of which we can determine the electron spin state.
Mapping Quantum Algorithms to Spin Qubit Architectures in Silicon
There are a number of small proof-of-principle quantum algorithms which can be considered important for Noisy Intermediate Scale Quantum devices. Many of these are relatively simple to understand, but still need to be implemented on physical devices. In doing so choices need to be made, such as which qubit in the algorithm matches to which physical qubit in the device, or which how the operations should be performed and optimised in order to achieve the best (lowest error) performance. This project will examine how to map small scale quantum algorithms and protocols to physical devices.
Quantum Algorithms on near-term physical devices
Quantum algorithms have been shown to provide a way of speeding up certain computationally demanding classical algorithms, across an extensive range of problem types, including optimisation, solving linear algebra problems, and factoring large numbers. In these projects we will examine two broad aspects of quantum algorithms: how best can quantum algorithms be formulated to run on near-term physical devices, specifically the silicon hardware being developed at SQC; what other potential real-world applications can quantum algorithms solve. Possible project areas include: the improvement of key quantum algorithm sub-routines, such as the quantum Fourier transform; the optimisation of workhorse quantum algorithms, such as quantum phase estimation; improvements to near-term heuristic quantum algorithms, such as QAOA (quantum approximate optimisation algorithm) and VQE (variational eigensolver); the application of quantum algorithms to industry relevant areas such as finance, transport, and chemistry.
Logical Qubit Using Atom Qubits in Silicon
After successful demonstration of multi-qubit quantum processors, we are now focusing on increasing qubit numbers and implementing quantum error correction. A logical qubit is a basic building block of a fault-tolerant quantum computer, where the logical states can be encoded using a collection of physical qubits to protect against errors. In this project, we will develop the logical qubit using an iterative development loop, where a prototype device is designed, fabricated, tested, and thoroughly characterised, so that the extracted device parameters can inform the design of the subsequent device. Being a full-stack company, Silicon Quantum Computing (SQC) has in-house expertise and is well equipped to execute rapid prototyping cycle. Consequently, we believe that our team at SQC is in a very strong position to integrate all of the individual components needed to realise a first ever logical qubit in silicon, bringing us closer to the transformative potential of quantum computing technology.
Classical Quantum Control Hardware and Embedded Software
The Embedded Systems team are responsible for the embedded software, FPGA firmware and RF/mixed signal hardware design for the quantum control system at SQC. Our control system is based around a large and high performance FPGA technology from AMD Xilinx, called the RFSOC. It has wideband ADCs and DACs operating at multi-GHz rates, to generate the control pulses required to operate our devices, and readback the spin of a single electron. We also work with the wiring, electronics and cold finger PCB design which are deployed inside cryogenic fridges. Get in touch if you're interested in any of these technologies.
-
My research group works in the broad area of theoretical condensed-matter physics with emphasis on nanomagnetism and spintronics. In particular, I focus on topics such as dynamics of skyrmions and other topological spin textures in ferro/antiferromagnets, spintronics with topological insulators, quantum phenomena in low-dimensional spin-orbit systems. The majority of my work either builds on observations made in recent experiments or predicts new phenomena that should be observable in the near future.
-
The research in our group is focused on unconventional magnetism. This includes topologically protected spin systems such as skyrmions, or novel multifunctional materials. For example, multiferroics feature a simultaneous electric polarization and magnetic ordering, and both properties can even be switched by each other. I.e., with an applied electric field the magnetic order can be changed and vice versa. This opens new opportunities for technological applications such as new computer hard drives or in spintronics. We are investigating these materials by SQUID magnetometry, optical Laser spectroscopy and neutron scattering, in order to obtain a deeper knowledge about the involved physics.
-
My team aims to better understand our own Milky Way Galaxy’s unique evolutionary history by comparing it to its extragalactic cousins. I am leading the GECKOS survey which uses the Very Large Telescope with MUSE - a Hyper Spectral Imaging or 3D spectroscopic instrument - that will provide a detailed cross-section of 35 galaxies with similar properties to our own Galaxy. With these 3D data cubes, we can determine the chemical composition of its stars and gas, with the side-on viewpoint giving us a chance to detect faint signatures that were left behind by small galaxy mergers a long time ago. There are three types of projects on offer that focus on either imaging data, 3D spectroscopy, or building mock observations from models. All projects are data intensive and involve Python programming, hence an entry-level of programming is preferred.